Fondazionetronchetti.it
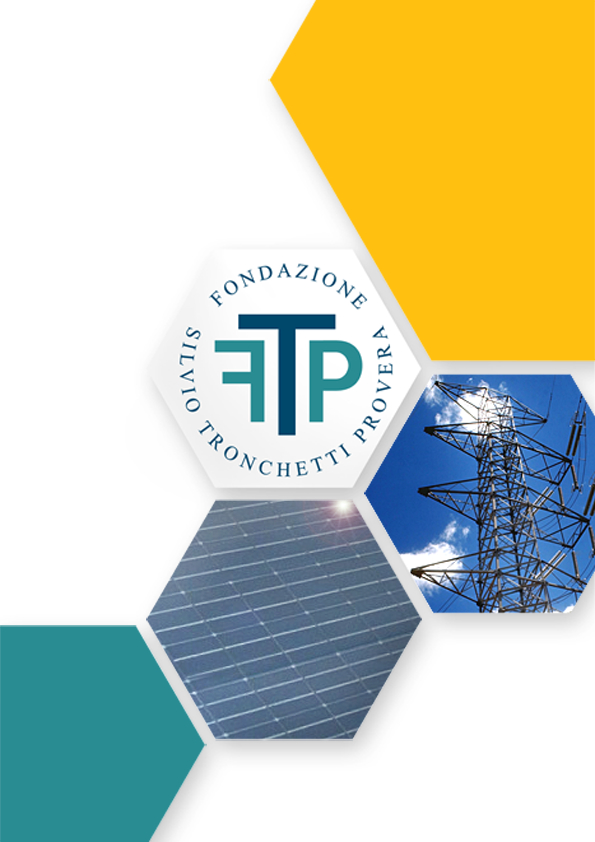
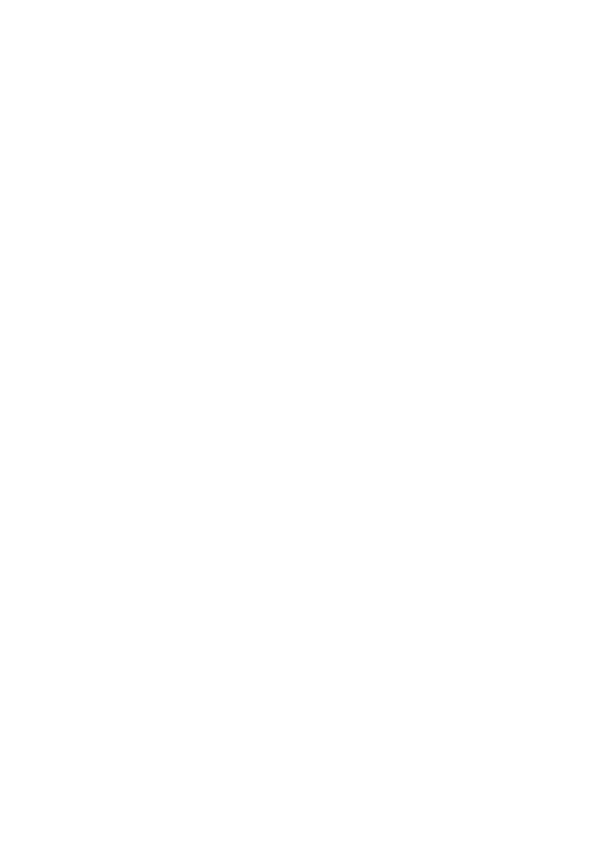
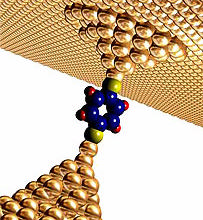
Hot Advance for Thermoelectrics
Cheap organic molecules could more efficiently convert waste heat into electricity.
Inside fossil-fuel and nuclear-power plants, a large share of energy in fuel is wasted as heat rather
than converted into electricity or mechanical power. But the search for a practical material that can
convert at least some of this waste heat into electricity has been long and frustrating.
Researchers have long known that some inorganic semiconductors can do this. Indeed, deep-space
probes have been powered by using such materials. But these inorganic materials are costly and
difficult to make, and have low efficiencies. Now, new research shows that certain organic
molecules produce voltage when exposed to heat. Ultimately, they could be much cheaper and thus
more practical to implement."This is the first demonstration that you can use organic molecules in
this kind of energy generation," says Rachel Segalman at the University of California, Berkeley,
who lead the latest work.
Experts had previously theorized that some organic molecules could have the qualities necessary to
generate electricity from heat. But until now, they lacked experimental proof, which the Berkeley
researchers were able to provide by isolating and measuring the properties of just a few molecules
of organic substances called benzene dithiols at a time. The researchers trapped a few molecules
between a sheet of gold and the ultrafine gold tip of a scanning tunneling microscope, which is so
sharp it can end in a single atom. They heated up the gold surface and measured, via the microscope
tip, the voltage that was created.
The experiments showed that the organic molecules have the three qualities that make for good thermoelectric materials. The first is the ability to create a voltage. But this works best when the materials have two other qualities: they do not conduct heat, but they do conduct electrons. That way, applying heat, rather than just raising the temperature of the material, actually drives electrons, creating a current. The results confirmed that the organic molecules could indeed be used to generate electricity from heat. Before they can be put to use, however, it will be important, Sales says, to design the molecules so that they arrange themselves between metal layers to make large-scale thermoelectric materials. What's more, so far the efficiency is very low, the researchers say. To improve this, they are creating and testing new versions of the molecules. "These are very simple molecules that the group is looking at," says J. Fraser Stoddart, at the University of California, Los Angeles. He's interested in the researchers' plans to alter the molecules to improve their thermoelectric properties. The research is only the first step, the researchers say, and, because much work remains, applications will be many years away.
Nanoparticles Help Create Cleaner Fuels
Bulk molybdenum disulphide (MoS2) is a ubiquitous, standard solid lubricant. However, extremely
small MoS2 nanoparticles have a potentially important application as a catalyst for producing
sulphur-free fuels. It is well known that material properties change when reducing particle sizes.
However, for MoS2 nanoparticles the size-dependent deviations from the bulk properties are more
pronounced than in other materials. Researchers at the Technical University Dresden and the
Forschungszentrum Dresden-Rossendorf have studied in detail the influence of the particle size on
the physical and chemical properties of MoS2. It was shown for the first time that not only the size,
but also the shape of the particle matters for the catalytic potential in fuel desulphurisation. The
results have been recently reported in Angewandte Chemie and Nature Nanotechnology.
Very small, sulphur-rich MoS2 nanoplatelets are well known as active catalysts for the
desulphurisation of fuels. It has recently been shown that the catalytic potential increases
dramatically with decreasing particle size. This effect has been correlated with the specific structure
along the edges of triangular nanoplatelets. In contrast to the semi-conducting bulk the edges of the
MoS2 nanoplatelets are electronically conducting and this is where sulphur-containing impurities in
the fuel are decomposed.
An international team of researchers from the Technical University Dresden, the
Forschungszentrum Dresden-Rossendorf, both in Germany, and at the Weizmann Institute in
Rehovot, Israel, have examined the properties of larger particles with many long and well accessible
edges. It was found that larger, regular three-dimensional particles promise a desulphurisation
potential that is similar to the nanoplatelets. Such particles have an octahedral form that is similar to
a bipyramid and require less effort in their production than the nanoplatelets that are ideally
synthesised directly on a gold support and cover it like a nanoconfetti.
For the first time, the research team has shown that the potential for the desulphurisation of fuels is
not limited to the smallest MoS2 particles, as the electronically conducting catalytic reaction sites
also occur in larger particles. Thus, the particle size and the three-dimensional structure crucially
determine the physical and chemical properties of MoS2 nanoparticles.
A joint theoretical and experimental investigation correlated the particle size and shape to the
structural and electronic properties that are responsible for the catalytic activity of MoS2
nanoparticles. Platelets, fullerenes or even nanotubes, MoS2 nanoparticles larger than 10
nanometres are semi-conducting like the bulk. In contrast, within a diameter range of 3 to 7
nanometres regular, three-dimensional structures occur that are composed of eight equilateral
triangles. Such particles have successfully been synthesised and observed experimentally by
transmission electron microscopy. For the edges and corners of such nano-octahedra the quantum-
mechanical calculations of the researchers from Dresden predict similar metallic properties to those
found in the catalytically active nanoplatelets. According to the model calculations, single-walled
nano-octahedra with a few hundred atoms are not stable. However, the observed multi-walled
particles of nested octahedra are predicted to be more stable species which promise similar catalytic
potential as the smaller nanoplatelets.
New Hydrogen Fuel System Promises A New Generation Of Renewable Energy
Thanks to research done by a University of Nevada, Reno professor in the area of hydrogen energy
generation, soaring power bills could become a thing of the past. And, finding a power source for
your car that costs as little as $1 per gallon could also soon become a welcome reality.
Manoranjan Misra, recently received a $3 million research grant from the U.S. Department of
Energy to continue his groundbreaking work in various forms of renewable energy. Misra's current
project focuses on harnessing photoactive material from the sun to generate hydrogen. Hydrogen is
one of the cleanest forms of energy, and studies have shown that it is 33 percent more efficient than
liquid fuels
Misra and his research team have created a new hydrogen material that has more than a billion
nanotubes, which gives it excellent potential to produce hydrogen from another abundant resource -
- water. Misra's small-scale hydrogen generation system, located in the Laxalt Mineral Research
Building, produces the material through an electrochemical process from applied ultrasonic waves.
"We are currently using simulated solar light in the lab," Misra said, "and we are finding our system
to be a good and robust way to facilitate the movement of electrons by the incident light to produce
hydrogen from water." By the end of the decade, Misra estimates that the system could grow to a
more industrial size scale, which would allow power companies to produce hydrogen that could be
used to power automobiles or power your home. The new power source is extremely cost-effective,
Misra says.
"What do we pay now for a tank of gas? A little less than three dollars per gallon? The equivalent
for hydrogen generation might be something more along the lines of $1 per gallon to produce,"
Misra says.
From farm waste to fuel tanks
Using corn waste as a starting material, researchers have created carbon briquettes with complex
nanopores capable of storing natural gas at 180 times their own volume and at one-seventh the
pressure of conventional natural gas tanks.
The breakthrough, announced by Pfeifer and colleagues at the University of Missouri-Columbia
(MU) and Midwest Research Institute (MRI) in Kansas City, is a significant step forward in the
effort to fit more vehicles to run on methane, an abundant fuel that is domestically produced and
cleaner burning than petrol. The technology has been incorporated into a test bed installed on a
pickup truck used by the Kansas City Office of Environmental Quality.
The briquettes are the first technology to meet the 180 to 1 storage to volume target set by the US
Department of Energy in 2000,
‘We are very excited about this breakthrough because it may lead to a flat and compact tank that
would fit under the floor of a passenger car, similar to current petrol tanks,' said Pfeifer. ‘Such a
technology would make natural gas a widely attractive alternative fuel for everyone.'
According to Pfeifer, the absence of such a flatbed tank has been the principal reason why natural
gas, which costs significantly less than petrol and diesel and burns more cleanly, is not yet widely
used as a fuel for vehicles.
Standard natural gas storage systems use high-pressure natural gas that has been compressed to a
pressure of 3600 pounds per square inch and bulky tanks that can take up the space of an entire car
trunk. The carbon briquettes contain networks of pores and channels that can hold methane at a high
density without the cost of extreme compression, ultimately storing the fuel at a pressure of only
500 pounds per square inch, the pressure found in natural gas pipelines.
The low pressure of 500 pounds per square inch is central for crafting the tank into any desired
shape, so ultimately, fuel storage tanks could be thin-walled, slim, rectangular structures affixed to
the underside of the car, not taking up room in the vehicle.
Pfeifer and his colleagues at MU and MRI discovered that that fractal pore spaces (spaces created
by repetition of similar patterns at different scales) are very efficient at storing natural gas.
‘Our project is the first time a carbon storage material has been made from corncobs, an abundantly
available waste product in the Midwest,' said Pfeifer. ‘The carbon briquettes are made from the
cobs that remain after the kernels have been harvested. The state of Missouri alone could supply the
raw material for more than 10 million cars per year. It would be a unique opportunity to bring corn
to the market for alternative fuels--corn kernels for ethanol production, and corncob for natural gas
tanks.'
The test pickup truck, part of a fleet of more than 200 natural gas vehicles operated by Kansas City,
has been in use since mid-October and the researchers are monitoring the technology's performance,
from mileage data to measurements of the stability of the briquettes.
In addition to efforts to commercialize the technology, the researchers are now focusing on the next
generation briquette, one that will store more natural gas and cost less to produce. Pfeifer believes
this next generation of briquette might even hold promise for storing hydrogen.
Nanoengineered concrete cuts CO2
Engineers at MIT are studying the nanostructure of concrete with a view to making a replacement
product that would not generate the same high carbon dioxide emissions.
The production of cement, the primary component of concrete, accounts for 5 to 10% of the world's
total carbon dioxide emissions, making it an important contributor to global warming.
The team found that the source of concrete's strength and durability lies in the organisation of its
nanoparticles, a discovery that could one day lead to a major reduction in carbon dioxide emissions
during manufacturing.‘If everything depends on the organisational structure of the nanoparticles
that make up concrete, rather than on the material itself, we can conceivably replace it with a
material that has concrete's other characteristics-strength, durability, mass availability and low cost,
but does not release so much CO2 into the atmosphere during manufacture,' said Franz-Josef Ulm.
If engineers could reduce carbon dioxide emissions in the world's cement manufacturing by even 10
per cent, that would accomplish one-fifth of the Kyoto Protocol goal of a 5.2 % reduction in total
carbon dioxide emissions. Ulm and his team studied the behavior of the nanostructure of cement.
They found that at the nano level, cement particles organise naturally into the most densely packed
structure possible for spherical objects.
Cement starts out as limestone and clay that are crushed to a powder and heated to 1500 °C in a kiln.
Most of the carbon dioxide emissions in this manufacturing process result from heating the kiln to a
temperature high enough to transfer energy into the powder. Ulm et al used a nano-indentation
technique to examine various hardened cement pastes with a nano-sized needle. An atomic force
microscope allowed them to see the nanostructure and judge the strength of each paste by
measuring indentations created by the needle.They discovered that the calcium-silicate-hydrate (C-
S-H) chemical bonding behaviour in concrete consistently displays a unique nanosignature, which
they call the material's genomic code. This indicates that the strength of cement paste, and thus of
concrete, does not lie in the specific mineral, but in the organisation of that mineral as packed
nanoparticles.
If the researchers can find or nanoengineer a different mineral to use in cement paste that has the
same packing density but does not require the high temperatures during production, they could
conceivably cut world carbon dioxide emissions by up to 10%.
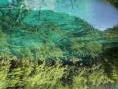
Making biodiesel from scum
Utah State University researchers have developed an approach that takes oil from pond scum and converts it to an algae-biodiesel fuel that could be commercially available by 2009.
Algae, a common variety of which is known as pond scum, can produce up to 38,000 litres of oil per acre and can be grown virtually anywhere. Most current biodiesel comes from soybean and corn oil. As supply and demand grows, so does the price of soybeans and corn. According to the researchers, people and animals rely on soybean and corn as a food commodity, eventually causing competition between commodities and growing enough product. Meeting the demand for biodiesel would require the world to use virtually all of its arable land, said Lance Seefeldt, USU professor of chemistry and biochemistry. Seefeldt, along with several fellow USU professors, formed the Biofuels Program to develop new and emerging technologies that will produce methane, biodiesel, hydrogen and alcohols from renewable, carbon-dioxide-neutral energy sources, such as consumer and agricultural waste and sunlight.
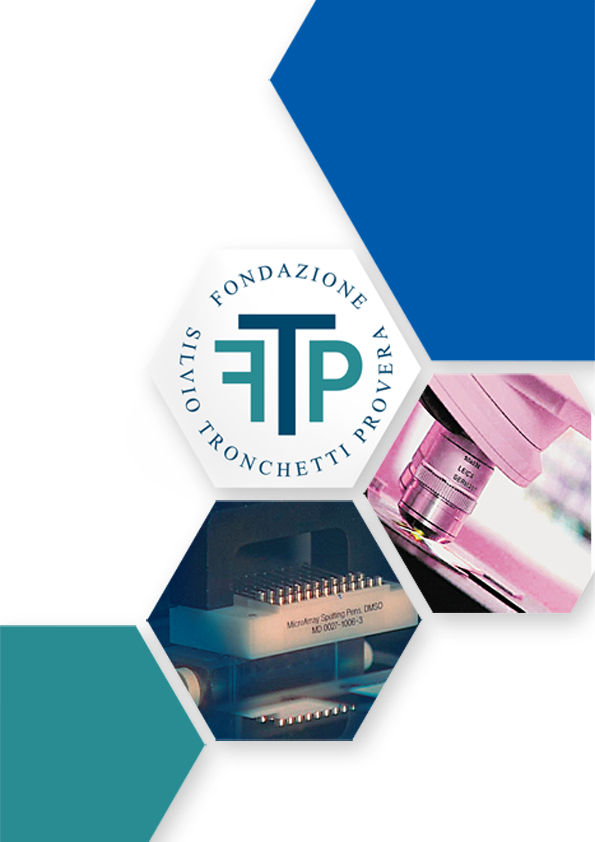
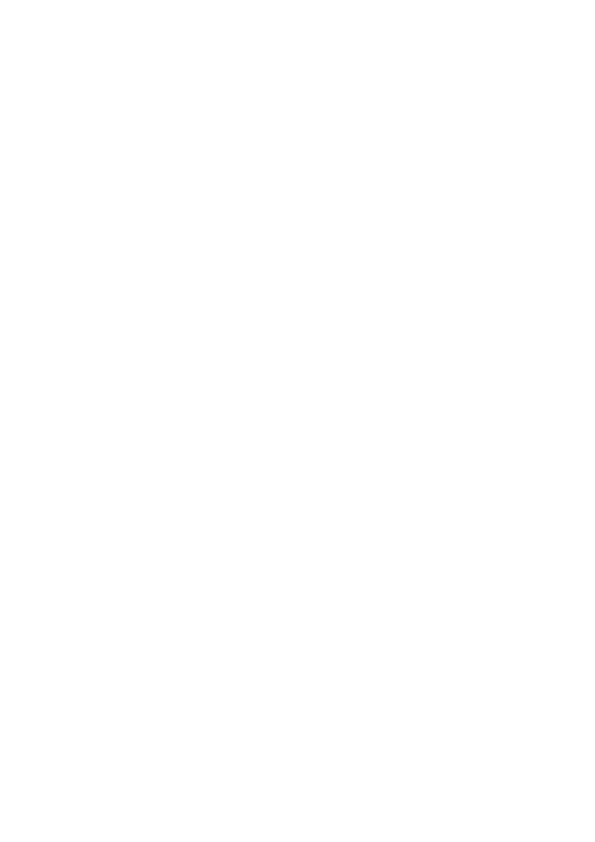
Cancer Is A Stem Cell Issue
There is an urgent reason to study stem cells: stem cells are at the heart of some, if not all, cancers.
Mounting evidence implicates a clutch of rogue stem cells brandishing ‘epigenetic' marks as the
main culprits in cancer.
A team in the Netherlands has uncovered a key protein that could stop these stem cells from
becoming malignant. "This is a hot topic in the cancer field," Maarten van Lohuizen of The
Netherlands Cancer Institute, "To be successful in cancer therapy you need to target these stem
cells: they are intrinsically resistant to chemotherapy."
Polycomb proteins have emerged as key players in cancer pathogenesis. They are powerful
epigenetic regulators that normally silence genes without altering the cell's DNA. Compounds that
regulate polycomb could result in novel anticancer drugs that shrink malignant tissue, and prevent
cancer recurrence, a common problem with most chemotherapies.
That tumours and stem cells have much in common has been known for many years. Both self-
renew and both spawn many different types of cells. But only recently, new techniques have
enabled biologists to identify stem cells buried in tumours.
Van Lohuizen has found that stem cells in cancerous tissues are locked in an immature state in
which they carry on multiplying instead of maturing into specific tissues. "Some resistant cancer
cells don't listen to the ‘stop' signal any more," he explains. That stop sign is delivered by the
polycomb proteins. They silence several genes at once by affecting the way the DNA is compacted
into chromatin fibres, without altering the DNA sequence.
Normally, the main role of the polycomb complex is to repress genes during development or when
stem cells are needed for tissue maintenance. But an aberrant polycomb spells trouble. In mice
where polycomb proteins have been genetically disabled, van Lohuizen has seen that the cells
become invasive and trigger cancerous growth. "This may be why gliomas are such lethal tumours,
because these stem cells become highly migratory," van Lohuizen points out.
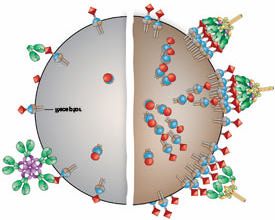
Strength In Numbers: low-affinity, multivalent interactions recruit antibodies to
kill cancer cells
Low-affinity, multivalent interactions can be more effective at killing cancer cells than high-affinity
interactions, according to research by Laura L. Kiessling and coworkers at the University of
Wisconsin
Bifunctional molecules (red and blue) target membrane receptors and recruit antibodies (green and purple) that kill
cells with high levels of receptors. Cancer cells (right) have more of the receptors than normal cells (left).
Nature uses a multitude of weaker interactions to distinguish one cell from another, Kiessling says. That way, if an agent interacts with the wrong type of cell initially, it easily can be displaced or dissociated. In contrast, most pharmaceutical targeting is based on a single high-affinity interaction. Kiessling and her coworkers target cancer cells with a small bifunctional molecule (ACS Chem. Biol.). One part of the molecule is a peptide mimic that binds tightly to cell-surface receptors known as avß3 integrins. The peptide mimic is attached to a carbohydrate called the a-Gal epitope. Human anti-Gal antibodies bind weakly to individual a-Gal epitopes but bind tightly when many are displayed together on the cell surface. "The integrin will be targeted with a high-affinity interaction, but the toxic agent is only recruited when you have the multivalent interaction," Kiessling says. Her group reported the synthesis of the molecule previously (ChemBioChem 2007, 8, 68). The researchers target the integrins because they are involved in the formation of new blood vessels. Integrins can be more abundant on cancer cells, and they are prevalent on the new blood vessels that tumors induce. "You don't need a receptor that's found solely on tumor cells," Kiessling says. "You just need one that's found in a significantly higher proportion" on tumor cells than on normal cells.
Ligands consisting of a peptide mimic (top) and a-Gal (bottom) target integrin receptors.
With the a-Gal-peptide combination, antibodies are recruited only to cells with high levels of integrins on the surface. If the a-Gal is replaced with the anticancer drug doxorubicin, the combination kills even normal cells, which generally have low levels of integrin on the surface compared with cancer cells. Thus, the doxorubicin conjugate is less selective for the cancer cells. The work is a "clever use of an inherent immune mechanism to exploit the fact that there are molecules that are more densely expressed on the surfaces of tumor cells than in normal tissues. Data from in vivo animal models would be needed to determine whether this approach has potential for diagnostic or therapeutic use in cancer patients." Kiessling is working with Paul Sondel, an oncologist and geneticist at UW Madison, to do in vivo studies to determine whether the strategy will work in animals. In addition, Kiessling is interested in using ligands that target two different cell-surface receptors to increase the selectivity for disease cells even more.
New Medical Technique Punches Holes In Cells, Could Treat Tumors
A large animal study has shown that certain microsecond electrical pulses can punch nanoscale
holes in the membranes of target cells without harming tissue scaffolding, including that in the
blood vessels - a potential breakthrough in minimally invasive surgical treatments of tumors.
The study on pigs, the first large animal trial for the irreversible electroporation (IRE) technique, is
described in the current issue of Technology in Cancer Research and Treatment.
IRE was developed at the University of California, Berkeley, which holds a number of patents on
the technology, by Ivan Rubinsky together with Gary Onik, Florida Hospital Celebration Health.
They founded Oncobionic two years ago to commercialize IRE.
It was first reported in the early 1970s that the application to cells of very fast electrical pulses - in
the microsecond and millisecond range - creates an electrical field that causes nanoscale pores to
open in the cell membrane. But research since then has mainly focused on reversible electroporation,
which uses voltages low enough to temporarily increase the cell membrane's permeability. The
holes in the cell membrane created by reversible electroporation close up shortly after treatment,
allowing the cell to survive."This concept of reversible electroporation really caught on in modern
biotechnology, especially over the last decade," said Rubinsky. "It is used primarily to help get
genes and drugs into cells. The field of irreversible electroporation was pretty much forgotten."
The researchers' work on irreversible electroporation is an outgrowth of studies done on a "bionic
chip" that Rubinsky and his UC Berkeley students were developing. The bionic chip merged living
cell tissue with electronic circuitry. In the course of understanding whether electroporation was
successful, the researchers discovered a range of electrical current that would cause permanent
damage to cell membranes without generating heat and thermal damage. Irreversible electroporation
uses electrical pulses that are slightly longer and stronger than reversible electroporation. With IRE,
the holes in the cell membrane do not reseal, causing the cell to lose its ability to maintain
homeostasis and die. The researchers say that IRE overcomes the limitations of current minimally
invasive surgical techniques that use extreme heat, such as hyperthermia or radiofrequency, or
extreme cold, such as cryosurgery, to destroy cells.They point out that temperature damage to cells
also causes structural damage to proteins and the surrounding connective tissue. For liver cancer,
the bile duct is at risk for damage. For prostate cancer, the urethra and surrounding nerve tissue is
often affected.
Electroporation, on the other hand, acts just on the cell membrane, leaving collagen fibers and other
vascular tissue structures intact. The researchers said that leaving the tissue's "scaffolding" in place
allows healthy cells to regrow far more quickly than if everything in the region was destroyed.
In the new study, the researchers set out to demonstrate that the IRE technique could produce
reliable and predictable results in a large animal model. They performed the IRE surgical technique
on 14 healthy female pigs under general anesthesia, using the same procedures as if the patients
were human.They used ultrasound imaging to guide the 18 gauge stainless steel electrodes to target
areas in the pigs' livers. The researchers applied 2,500 volts in eight 100-microsecond pulses spaced
100 microseconds apart to create lesions in the livers. They found that the lesions were immediately
apparent as dark spots on the ultrasound images, giving real-time feedback during the procedure.
The livers were then examined 24 hours, three days, seven days and 14 days after surgery."All of
the vessels, down to the microvasculature, remain intact with IRE treatment, so the healing process
is amazing," said Onik, who performed the surgery for the study. "Where it might take a year for a
cryosurgery lesion to resolve, IRE lesions resolved in two weeks. That has major implications in
terms of monitoring what you're doing and knowing that the cancer has been killed."
Another chronic drawback of heat or cryo treatments for cancer is the difficulty in treating cells that
are immediately adjacent to the blood vessels. Because blood maintains a relatively stable
temperature, it actually transfers heat or cold away from a treatment area in an attempt to return the
region to a normal temperature range. That means some cancerous cells might actually survive treatment."That counts for a lot of failures when treating liver cancers," said Onik. "With IRE, you can destroy cancerous cells right next to the blood vessels. It's a more complete treatment. In my clinical experience, this is about as good as it gets. We've been using other techniques for a long time. This provides significant improvements over other treatments." Onik does sound a note of caution, however. "While we are obviously very excited about this advance in tumor ablation, we are in the early stages of our learning curve," he said. "Experience developing cryosurgical ablation has taught us that we undoubtedly have much more to learn, and there is always the potential for unexpected results." Although the tissue in this study was healthy, the researchers found in a prior cell culture study that IRE effectively kills human liver cancer tissue. The IRE technology was cleared for human use by the U.S. Food and Drug Administration in November 2006. Onik is scheduled to begin human clinical trials for IRE this summer.
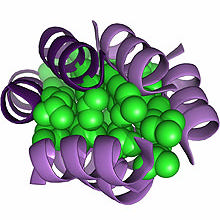
Protein Drugs with More Power
Scientists are learning to control a class of proteins that could have greater staying power in
the body.
A representation of the 3-D structure of a novel peptide
Yale University researchers have taken a step toward controlling the structure of an unusual class of proteins called beta-peptides. Eventually, these peptides could become the basis for drugs that are cheaper to manufacture than existing protein-based pharmaceuticals and last longer in the body. Most protein drugs in use and in development, such as antibodies that target cancer cells, are made from alpha-amino acids, which are the building blocks of naturally occurring proteins. But metabolic processes in the body's cells also produce strands of beta-amino acids, which differ from alphas in that they have one extra carbon atom. These beta-peptides are metabolized much more slowly than alpha-proteins. Moreover, proteins functions are to a large degree dependent on their structure; for example, some have pockets that fit another molecule the way a lock fits a key. Before researchers can make beta-peptides into drugs, however, they must be able to control their three-dimensional structure. Researchers have been working on this problem for about a decade, but previous researchers hadn't been able to keep beta-peptides from clumping together. Schepartz's group was able to make discrete, self-assembling "bundles" of the peptides. These bundle structures are similar to the structure of proteins made by the body. Beta-peptides can probably do all the same things as the proteins the body normally makes. But if researchers are able to reliably manufacture them, beta-peptides could have advantages over conventional protein-based drugs. Strings of alpha-amino acids must be relatively long before they can fold into a three-dimensional structure, but strings of beta-amino acids are able to do so at shorter lengths. Thus, these peptides could be less expensive to manufacture; as Schepartz says, they can "encode function in a smaller package" and therefore would require less starting material. Another advantage stems from the slower breakdown of beta-peptides in the body. Protein drugs made of alpha-amino acids are easily recognized by the body and metabolized quickly--sometimes too quickly to perform their function. They also cannot be taken orally, because enzymes in the stomach would degrade them. Beta-peptides aren't readily recognized by the body's enzymes, so drugs based on them would last longer and could probably be taken orally.
Silicon Medicines May Be Effective In Humans
As carbon-based life forms, humans and other animals, invariably, are treated for disease with the
help of carbon-based medicines. But now, in a promising new study, scientists have shown that
silicon - the stuff of computer chips, glass and pottery - may have extraordinary therapeutic value
for treating human disease.
"All medicines are carbon-based - like we are," says Robert West, a University of Wisconsin-
Madison chemist and one of the world's leading authorities on silicon chemistry. "There are about
50,000 biologically active molecules, and they're all mainly carbon-based."
But now, West and his colleagues report in the January edition of the journal Silicon Chemistry that
the effectiveness and safety of an important anti-inflammatory and anti-cancer drug were enhanced
remarkably by replacing one of the molecule's carbon atoms with a silicon atom.
The drug, indomethacin, is used to treat arthritis and some cancers, but the drug is little used
because it is quite toxic. "Our thought was, maybe we could tame it," says West. The idea,
according to West and colleague Galina Bikzhanova, was to make a simple chemical change to see
if the biological properties of the drug could be modified to be safer and more effective. By trading
one of the drug's carbon atoms for a silicon atom and exposing the modified agent to cancer cells in
culture, West's group found that the molecule's effectiveness was enhanced and its toxicity greatly
reduced.
Indomethacin is an anti-inflammatory drug belonging to a family of compounds known as COX
inhibitors. COX inhibitors selectively block an enzyme that causes pain and swelling. "Our
molecule is a COX-2 inhibitor like Tylenol and Vioxx and other such drugs," notes West.
How the drug and other COX inhibitors work against cancer is not well understood, but they clearly
demonstrate anti-cancer properties, according to West. The modified molecule with the silicon atom
was exposed to several types of cancer cells in culture in the new study. The agent had significant
effects on skin and prostate cancer cells, but showed the greatest efficacy against pancreatic cancer
cells. "The results are very promising, especially in using our compound in combination with
standard anticancer drugs," says West, who conducted the study in collaboration with Bikzhanova,
Irina Toulokhonova of UW-Madison and Stephen Gately of RND Pharmaceuticals in Scottsdale,
Ariz. Tested on cancer cells in cultures, the modified drug both slowed the growth of cancer cells
and killed cancer cells directly. The high activity was against pancreatic cancer cells. This is
important because pancreatic cancer responds poorly to any therapy and is almost always fatal
because it is hard to detect and spreads rapidly.
Perhaps just as important, the silicon-modified molecule exhibited far less toxicity than the all-
carbon-based form of the drug. "We tested for toxicity and it is well tolerated," West explains.
"That's really different than indomethacin and the other indomethacin derivatives that have been
made." West and his colleagues made four variants of the drug, each with a slightly different
chemical structure but all containing the silicon atom.
Why does silicon have this effect? "It changes the property of the molecule, but not drastically," the
Wisconsin chemist explains. "It's fine tuning," says Bikzhanova. "We don't know why, but it led to
this unexpectedly strong effect against cancer cells."
Using Nano-magnets To Enhance Medical Imaging
Nanoscale magnets in the form of iron-containing molecules might be used to improve the contrast
between healthy and diseased tissue in magnetic resonance imaging (MRI)--as long as the
concentration of nanomagnets is carefully managed--according to a new report by researchers at the
National Institute of Standards and Technology (NIST) and collaborators. Molecular nanomagnets
are a new class of MRI contrast agents that may offer significant advantages, such as versatility in
design, over the compounds used today.
This test image shows what happens when nanomagnets are used to alter the nuclear properties of hydrogen in water,
increasing brightness (bright spots below left and center) compared to deionized water (above)
Contrast agents are used to highlight different tissues in the body or to help distinguish between healthy and diseased tissue. NIST is working with two universities and a hospital to design, produce and test nanomolecules that might make MRI imaging more powerful and easier to perform. The new paper resolves a debate in the literature by showing that iron-containing magnets just two nanometers wide, dissolved in water, do provide reasonable contrast in non-clinical MRI images--as long as the nanomagnet concentration is below a certain threshold. (A nanometer is one billionth of a meter.) Previous studies by other research groups had reached conflicting conclusions on the utility of molecular nanomagnets for MRI, but without accounting for concentration. NIST scientists, making novel magnetic measurements, were able to monitor the molecules' decomposition and magnetic properties as the composition was varied. The injectable dyes currently used as MRI contrast agents are of two types. Magnetic ions, which alter the nuclear properties of hydrogen in water, offer the advantage of consistent identical design but provide low contrast. The second category encompasses particles of thousands of atoms or crystals, which alter local magnetic fields; they provide contrast variation in a larger region but have irregular designs and magnetic properties that are difficult to control. By comparison, molecular nanomagnets can be designed to have consistent properties and high contrast. In addition, they might be modified to act as "smart" materials whose contrast could be turned on only when bonded to a target molecule or cell. Toxicity is not believed to be an issue, because iron is naturally found in the body and other studies have found that these materials are non-toxic at the concentrations used in MRI. NIST works with Florida State University to make single-molecule magnets less than five nanometers (nm) in diameter, and works with the University of Colorado at Boulder to make nanocrystals in the 10-50 nm range. The agency is pioneering methods for manipulating and measuring the magnetic properties of these compounds and is developing instrumentation for understanding how contrast agents work and how to control contrast properties. Researchers correlate the measured properties to the observed MRI response under non-clinical conditions using imagers at The Children's Hospital in Denver. The information gained is fed back into recipes for making even better nanomagnets.
Source: http://www.fondazionetronchetti.it/new/img/pdf/1436879226_news02_07b.pdf
Codigo Monetario y Financiero de la Republica Dominicana Santo Domingo, Rep. Dom. Agosto 2007 Título I: Marco Regulatorio e Institucional . 7 Sección I: Principios de la Regulación del Sistema Monetario y Financiero .7Sección II: Organización de la Administración Monetaria y Financiera .13Sección III:
Serving People with Developmental Disabilities since 1978 on a World-renowned saxophonist Branford Marsalis made a very special appearance at the newly renovated Kodak Hall at Eastman Theatre to receive the 13th Annual Lifetime Inspiration Award. The award, bestowed annually by the Lifetime Assistance Foundation, was presented to Branford Marsalis by WXXI's Thomas Hampson and Patrick Burke, Regional President of First Niagara Financial Group and Chairman of the Foundation Board.