Anodic oxidation of nitrobenzene on bdd electrode: variable effects and mechanisms of degradation
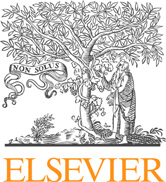
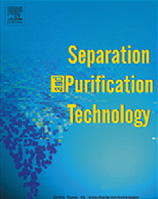
Contents lists available at
Separation and Purification Technology
Anodic oxidation of nitrobenzene on BDD electrode: Variable effectsand mechanisms of degradation
Nejmeddine Rabaaoui ,Younes Moussaoui ,Mohamed Salah Allagui , Bedoui Ahmed ,Elimame Elaloui
a Materials, Environment and Energy Laboratory, Science Faculty of Gafsa, University of Gafsa, Gafsa 2112, Tunisiab Science Faculty of Gafsa, University of Gafsa, Tunisiac Science Faculty of Gabes, University of Gabes, Gabes 6072, Tunisiad Physical Organic Chemistry Laboratory, Science Faculty of Sfax, University of Sfax, Tunisia
The electrochemical oxidation of pesticide, nitrobenzene (NB) as one kind of pesticide that is potentially
Received 5 December 2012
dangerous and biorefractory, was studied by galvanostatic electrolysis using boron-doped diamond
Received in revised form 27 January 2013
(BDD) as anode. The influence of several operating parameters, such as applied current density, support-
Accepted 28 January 2013
ing electrolyte, and initial pH value, was investigated. The best degradation occurred in the presence of
Available online 5 February 2013
Na2SO4 (0.05 M) as conductive electrolyte. After 8 h, nearly complete degradation of nitrobenzene wasachieved (88%) using BDD electrodes at pH = 3 and at current density equals 60 mA cm2. The decay
kinetics of nitrobenzene follows a pseudo-first-order reaction. Aromatic intermediates such as phenol,
catechol, resorcinol, 1,2,4-trihydroxybenzene, hydroquinone and benzoquinone and carboxylic acids
such as maleic glycolic, malonic, glyoxilic and oxalic, have been identified and followed during the nitro-
benzene treatment by chromatographic techniques. From these anodic oxidation by-products, a plausible
Wastewater treatment
reaction sequence for NB mineralization on BDD anodes is proposed.
Ó 2013 Elsevier B.V. All rights reserved.
H2O2/UV and H2O2/Fe2+/UV . The great effectiveness of AOPsis due to the production of hydroxyl radical (OH), which is a non-
Nitrobenzene belong to nitroaromatic compounds which are
selective, very powerful oxidizing agent able to react with organics
deemed to be the exclusive pollutants from anthropogenic sources
giving dehydrogenated or hydroxylated derivatives, up to their
. These chemicals are widely spread across the environment
complete mineralization (conversion into CO2, water and inorganic
due to their extensive use as raw materials or synthetic intermedi-
ates in the manufacture of pharmaceuticals, wood preservatives,
In the last years, effective electrochemical treatments for the
rubber chemicals, pigments, dyes, plastics, pesticides and fungi-
destruction of biorefractory organics in waters are being devel-
cidal agents, explosives and industrial solvents o-Nitrophe-
oped. The most usual technique is anodic oxidation, where solu-
nols pose potential risks to both the human health and the
tions are decontaminated during electrolysis by the direct
ecosystem since they are toxic to plants, fish and many other
reaction of pollutants with adsorbed OH formed at the anode sur-
organisms and can accumulate in the food chain The US Envi-
face from oxidation either of water in acid and neutral media or
ronmental Protection Agency (USEPA) has classified o-nitrophenol
hydroxide ion at pH P 10
as a pollutant of group C (possible human carcinogens) . Purifi-cation of wastewater polluted by nitrobenzene is a very difficult
H2O ! OHads þ Hþ þ e
task. The presence of nitro group in the aromatic ring enhancesthe stability of these molecules to chemical and biological degrada-
OH ! OHads þ e
tion . In order to treat these toxic effluents, several papershave reported the rapid removal of nitro-aromatic from waters
However, most aromatics in acid and alkaline media treated by
by some advanced oxidation processes (AOPs), such as O
anodic oxidation with conventional anodes such as Pt, PbO
PbO2, doped SnO2 and IrO2, are slowly depolluted due to the gen-eration of difficulty oxidizable carboxylic acids The recent
⇑ Corresponding author at: Materials, Environment and Energy Laboratory,
use of a boron-doped diamond thin film anode has shown that it
Science Faculty of Gafsa, University of Gafsa, Gafsa 2112, Tunisia. Tel.: +216 28153 135; fax: +216 76 677 441.
has much larger O2 overvoltage than the above anodes, giving a
E-mail address: (N. Rabaaoui).
much higher concentration of adsorbed OH and a quicker oxida-
1383-5866/$ - see front matter Ó 2013 Elsevier B.V. All rights reserved.
N. Rabaaoui et al. / Separation and Purification Technology 107 (2013) 318–323
tion of pollutants. Anodic oxidation with BDD then seems a suit-
potentiostat–galvanostat. The solution pH was measured with a
able method for degrading organics up to their total mineraliza-
Crison 2000 pH-meter. Total Organic Carbon (TOC) amounts in
tion, as found for HClO4 aqueous solutions containing carboxylic
aqueous solutions were obtained with a Shimadzu VCSH carbon
acids such as acetic, malic, formic and oxalic indigo carmine
analyzer. During all experiments, samples were collected and
phenol and 2-(4-chloro-2-methylphenoxy) propionic
immediately measured without using any filter. Chemical oxygen
acid aromatic amines and for amarantha dyestuff in Na2-
demand (COD) data were obtained with a Merck Model SQ118
spectrophotometer after digestion of samples in a Merck Model
Our research focused on studying the degradation rate of nitro-
TR-300 thermoreactor. nitrobenzene and all other intermediates
benzene effluents using BDD electrode. A concentrated (2 mM)
were identified by reversed-phase chromatography with a water
solution of this pollutant was treated to explain the role of aro-
system composed of a Waters 600 HPLC liquid chromatography fit-
matic intermediates and carboxylic acids formed on the degrada-
ted with a Spherisorb ODS2 5 lm, 150 4.6 mm column, at room
tion process. The decay kinetics of nitrobenzene and the
temperature, and coupled with a waters 996 photodiode array
evolution of by-products for nitrobenzene were then followed by
detector selected at 256 nm, controlled through a Millennium-32
chromatographic techniques, allowing the proposal of a reaction
programÒ. These analyses were made by injecting 20 lL aliquots
pathway for nitrobenzene mineralization.
into the chromatograph and circulating a 39/59/2 (v/v/v) metha-nol/water/acetic acid mixture at 0.7 mL min1 as mobile phase.
Generated carboxylic acids were followed by ion-exclusion chro-
2. Materials and methods
matography by injecting 20 lL samples into the above HPLC sys-tem with a SUPELCOGEL C-610H, 250 4.6 mm column, at 35 °C
2.1. Electrodes preparation
from Bio-Rad. For these measurements, the photodiode detector(L-2400) was selected at 210 nm and the mobile phase was
PbO2 was deposited galvanostatically on the pretreated lead
substrate by electrochemical anodization of lead in oxalic acid
2SO4 at 0.2 mL min1. Inorganic ions were analyzed by
ion chromatography using a Dionex ICS-1000 Basic Ion Chromatog-
solution (100 g L1) at 25 °C. This acid solution was electrolyzed
raphy system fitted with an IonPac AS4A-SC, 25 cm 4 mm anion-
galvanostatically for 30 min at ambient temperature using an ano-
exchange column. These measurements were conducted by inject-
dic current density of 100 mA cm2. The cathode was stainless
ing 25 lL samples and using a mobile phase composed of 1.8 mM
steel (austenitic type), the two electrodes were concentric with
of sodium carbonate and 1.7 mM of sodium bicarbonate at the flow
the lead electrode as axial. This arrangement gave the formation
rate of 0.8 mL min1.
of a regular and uniform deposit .
BDD films were provided by CSEM and synthesized on a con-
ductive p-Si substrate (1 mm, Siltronix) via a hot filament, chemi-
3. Results and discussion
cal vapor deposition technique (HF-CVD). The temperature of thefilament was from 2440 to 2560 °C and that of the substrate was
3.1. Effect of experimental parameters on nitrobenzene mineralization
monitored at 830 °C. The reactive gas used was 1% methane inhydrogen containing 1–3 ppm of trimethylboron. The gas mixture
3.1.1. Effect of the anode material
was supplied to the reaction chamber at a flow rate of 5 L min1 to
A series of comparative electrolyses for nitrobenzene was per-
give a growth rate of 0.24 lm h1 for the diamond layer. This pro-
formed using the BDD anode. Solutions containing 256 mg L1
cedure gave a columnar, randomly textured, polycrystalline dia-
COD of each nitrophenol of pH 3.0 were treated at 60 mA cm2
mond film, with a thickness of about 1 lm and a resistivity of
and at 20 °C by prolonging the electrolysis time to attain almost
15 mX cm (±30%) onto the conductive p-Si substrate .
overall decontamination. In these trials the solution pH remainedpractically constant and the starting colorless solutions changed
2.2. Electrolysis of nitrobenzene solutions
to a clear brown color after 1 h of electrolysis, becoming colorlessagain at the end of treatment. This change in solution color can be
Galvanostatic electrolyses were carried out at BDD and PbO
related to the formation of benzoquinone intermediates, as will be
electrodes, with current density ranging from 0 to 60 mA cm2.
discussed below.
Runs were performed at 20 °C. Solutions of 256 mg L1 of pure
The COD abatement as function of the consumed specific charge
nitrobenzene were used. Electrolysis was done with 0.05 M of dif-
obtained for the above experiments is depicted in . A fast and
ferent types of electrolytes NaCl, KCl, Na
quite COD decay can be observed for our solution, yielding more
3PO4 and Na2SO4 with pH
around 3.0–10.0. All electrolyses were conducted in an open, one-compartment and thermostated cylindrical cell containing a
150 ml solution stirred with a magnetic bar. The anode was a
nitrobenzene by PbO2"
42 cm2 BDD. For comparative purposes, a 42 cm2 PbO2 was alsoemployed as anode. The cathode was always a 42 cm2 graphite
nitrobenzene by BDD
bar from Sofacel. The interelectrode gap was about 3 cm. The cur-rent and potential measurements were carried out using digital
COD / mgL 100
2.3. Analytical techniques
The used compounds were either reagent or analytical grade
from Sigma–Aldrich. Anhydrous sodium sulfate used as back-
ground electrolyte was analytical grade from Fluka. All solutions
were prepared with water from a Millipore Milli-Q system (con-
Fig. 1. Chemical oxygen demand removal for the anodic oxidation of 100 mL of
ductivity <6 108 S cm1). The temperature of the electrolyte
solution with 2 mM of nitrobenzene in 0.05 M Na2SO4 of pH 3.0. (h) BDD or (j)
was fixed by using a water thermostat. The current density for
PbO2 anode and a graphite cathode (all them of 42 cm2 area) operating at
the electrolysis was kept at the desired level with an Amel 2053
60 mA cm2; pH = 3 and at T = 20 °C.
N. Rabaaoui et al. / Separation and Purification Technology 107 (2013) 318–323
than 92% mineralization after consumption of 20 A h L1 for 8 h.
ven charge loading. It was found that the amount of polymeric
These findings evidence that nitrobenzene degradation process
products increased with a rise in current density.
on BDD since BDD (OH) formed from water oxidation by reaction
The experimental results above suggest that the usage of differ-
is able to completely mineralize these compounds at similar
ent current densities at different oxidation stages is a good practice
in industrial applications.
In order to compare the oxidation ability of BDD and PbO2, a
commercially and more available material, we decided to investi-
3.1.3. Effect of pH value
gate the degradation of nitrobenzene using the latter anode. In this
Solution pH is an important factor for wastewater treatment. In
case, the effluent acquired an intense dark brown color and a black
anodic oxidation, there are many reports on the influence of solu-
precipitate, probably composed of polymeric products, was slowly
tion pH, but the results are diverse and even contradictory due to
formed during the first hour of electrolysis. As illustrated in
different organic structures and electrode materials Some
the use of PbO2 leads to a slow COD decay, only attaining 71%
authors reported that the oxidation process is more favorable in
decontamination of the nitrobenzene effluent 20 A h L1 for 8 h.
acidic media . In contrast, others indicated that the efficiencyof the process was increased in alkaline media . According to
3.1.2. Effect of current density
this literature, it can be concluded that the effect of pH strongly de-
Applied current density is an important factor affecting the
pends on the nature of the investigated organics and of the sup-
electrolysis kinetics and process economics. It corresponds to the
porting electrolyte. Therefore, the effect of pH on the degradation
ratio between the applied current and the surface of the working
rate of NB was studied at large pH range from acidic to basic. Aque-
electrode. Therefore, the current density can be altered by chang-
ous solutions of NB (246 mg L1) were electrolyzed at pH values of
ing the current and/or the surface of the working electrode. The
3, 6, and 10 (). As can be seen from this figure, the degradation
anodic oxidation process is an electrochemical process ruled by
of NB in the acid medium is more efficient than the degradation in
the following equation:
alkaline and neutral medium. This effect may be due to the exten-sive oxidation and/or chemical modification of the electrode sur-
BDD ! ðH2OÞ ! BDD ðOHÞ þ Hþ þ e
face, which suggests a change in the surface properties.
Moreover, the solution was not buffered at the working pH value,
So, the current increases and improves the degradation rate by
the latter progressively decreased during the reaction presumably
the rise of the OH production rate, as shows. This latter dem-
due to the formation of oxidized by-products. In fact, COD reduc-
onstrates two different regions: the first is when the charge load-
tion attains more than 92% at pH = 3.0 with a total disappearance
ing is below 3 A h L1. In this case, the COD, decreasing linearly,
of the color but it does not exceed 60% at pH = 10.0. This is very
is always more rapidly reduced when current density rises from
important because industrial wastewater may have different pH
20 to 60 mA cm2 and there is no substantial effect of the current
density on the oxidation efficiency. Note that the current efficiency
In pH = 10.0, we observe that the solutions become a little bit
(CE) is close to 100%, independent of the current densities used.
turbid after oxidation. This indicates the formation of polymeric
The second is when the charge loading is above 3 A h L1 In this
intermediate products by the hydroxyl group of NB that makes
case, the COD decreases when current density rises from 20 to
the degradation much more difficult
The reactions of anodic oxidation of pollutants occur heteroge-
neously considering that pollutants must be transported to the
3.1.4. Effect of type of electrolyte
electrode surface first, and then be oxidized there. The pollutant
shows the influence of nature of supporting electrolyte
degradation may be subjected to current control or mass transfer
(NaCl, KCl, Na2SO4 and Na3PO4) on the evolution with Q of COD
control. Initially, the COD concentration was relatively large, and
during galvanostatic electrolyses (j = 60 mA cm2) of 2 mM of
accordingly its reduction rate was subjected to the current control.
nitrobenzene at 20 °C. It can be seen that the complete mineraliza-
In this case, 100% CE could be obtained and COD decreased linearly
tion of organic matter is achieved in the presence of the four elec-
with the charge loading based on the mathematical model devel-
trolytes. The graph shows that the abatement of the COD of
oped by Comninellis and coworkers In the case of the
nitrobenzene in the presence of Na2SO4 or Na3PO4 is more rapid
COD reduction rate that was subjected to the mass transfer control,
than in the presence of NaCl and KCl. This suggests that the oxida-
only part of current supplied was used to oxidize pollutants, while
tion of NB should be carried out by both direct electro-oxidation
the rest current was wasted for generation of oxygen. Therefore,
and mediated oxidation by hydroxyl radicals and other strong oxi-
the residual COD increased as the current density increased at a gi-
dants electro-generated from the oxidation of the supporting elec-
Fig. 2. Influence of applied current density on the evolution of COD with specific
Fig. 3. Influence of pH values on the evolution of COD with specific electrical charge
electrical charge passed during electrolysis of nitrobenzene on BDD anode: (d)
passed during electrolysis of nitrobenzene on BDD anode: (j) pH = 3, (N) pH = 6,
j = 20 mA cm2, (N) 40 mA cm2, and (j) 60 mA cm2. Conditions: initial concen-
and (d) pH = 10. Conditions: initial concentration 2 mM; current density:
tration 2 mM; electrolyte 0.05 M Na2SO4; pH = 3 and at T = 20 °C.
j = 60 mA cm2; Electrolyte 0.05 M Na2SO4 and at T = 20 °C.
N. Rabaaoui et al. / Separation and Purification Technology 107 (2013) 318–323
sorbed on the PbO2 surface, where it reacts selectively and more
slowly with adsorbed organics According to this behavior,
several authors recently reported that phenol, o-substituted phe-
nols and pesticide methamidophos are more rapidly destroyed
with BDD than with PbO
2 using small conventional electrolytic
The above concentration decays were well-fitted to a pseudo
first-order kinetic equation and the excellent straight lines thus ob-tained are depicted in the inset panel of . This suggests the
production of a constant OH concentration at each anode during
electrolysis, which is much higher than that of the initial pollutant
reacting with it either on the PbO2 surface or in the vicinity of the
Fig. 4. Influence of supporting electrolyte on the evolution of COD with specific
electrical charge passed during galvanostatic electrolyses of nitrobenzene on BDDanode: (r) KCl 0.05 M, (d) NaCl 0.05 M, (j) Na2SO4 0.05 M, and (N) Na3PO4 0.05 M;
3.3. Identification and evolution of intermediates
conditions: initial concentration: 2 mM; pH = 3; current density: j = 60 mA cm2and at T = 20 °C.
Mineralization of aqueous NB solution was then monitored by
measuring the TOC values during treatment. The decay of the
trolyte. It seems that the mediated oxidation by the electro-gener-
TOC as a function of specific electric charge is shown in
ated reagents from the anodic oxidation of salt plays an important
The overall mineralization was attained after 480 min showing
role in the efficiency of the electrochemical process. To explain
that electrogenerated hydroxyl radicals efficiently destroy aro-
these results, it has to be taken into account that BDD-anodic oxi-
matic hydroxylated intermediates and all final carboxylic acids as
dation of aqueous solutions containing chloride, sulfate and phos-
phate anions promotes the formation of hypochlorites, persulfates
The degradation of the toxic organic compounds by the anodic
and perphosphates respectively, and that these chemical species
oxidation process can produce some aromatic intermediates that
are very powerful oxidant with high standard reduction potentials
can be much more toxic than the initial product and that can pol-
This can be explained assuming that the oxidation with per-
lute not only the water but also the environment. Thus the identi-
sulfates or perphosphates seems to be more severe in the final
fication of these intermediates is necessary to be able to propose a
stages and it promotes the formation of carbon dioxide but the oxi-
mechanism of mineralization by the attack of hydroxyl radicals
dation with hypochlorites lead to a less effective oxidation of NB
and in this case only mediated oxidation by hydroxyl radicals
During anodic oxidation of NB, main reactions are successively
should be considered in the mechanism of electrochemical process
electrophilic addition of hydroxyl radical on the aromatic ring
leading to the formation of polyhydroxylated benzene derivatives,such as phenol, catechol, resorcinol, 1,2,4-trihydroxybenzene,
3.2. Decay kinetics of nitrobenzene
hydroquinone and benzoquinone. The evolution of these sub-stances in aqueous medium is represented in in that these
The kinetics of the reaction of initial pollutants with BDD (OH)
compounds are accumulated to the maximum between 120 min
and 180 min of treatment with BDD, further being slowly removed
2 (OH) under the above operating conditions was followed
by reversed-phase HPLC. The change of concentration of nitroben-
to disappear in 480 min, that is a time similar to that needed for
zene with electrolysis time is given in . The time required for
the total removal of the initial pollutant (see
total removal of nitrobenzene with BDD is very similar to that
The evolution of carboxylic acids which are identified during
needed for their overall mineralization, indicating that nitroben-
the mineralization of NB (malonic, glyoxilic and oxalic) is repre-
zene persist in solution up to the end of the combustion process
sented in where they start to form at the beginning of the
and they are degraded in parallel to their by-products by BDD
electrolysis and they attain maximum concentration between 60
(OH). However, shows that nitrobenzene appears to be de-
and 120 min with a similar kinetic. Maleic and glycolic acids were
stroyed at slower rate using a PbO
detected in low concentrations The higher concentration is attrib-
2 anode and completely removed
in a time much more than 480 min. It is well known that OH
uted to glyoxilic acid: 3.2 mg L1 at 60 min. Then the concentration
formed at BDD remains mainly free on its surface where it attacks
of these acids decreases to be eliminated after 480 min which is
non-selectively and directly organics, whereas this radical is ad-
proved in where the TOC is removed in the same period.
60 120 180 240 300 360 420
Time / min
120 180 240 300 360 420 480
Time/ min
Time/ min
Fig. 5. Decay of nitrobenzene concentrations with electrolysis time for the trials
Fig. 6. TOC removal with time during anodic oxidation of nitrobenzene aqueous
reported in The inset panel shows the kinetic analysis for the corresponding
solution on BDD anode: conditions: initial concentration: 2 mM, current density:
experiments assuming a pseudo-first-order reaction for each initial compound.
j = 60 mA cm2; electrolyte 0.05 M Na2SO4; pH = 3 and at T = 20 °C.
N. Rabaaoui et al. / Separation and Purification Technology 107 (2013) 318–323
o
C 0 0 60 120 180 240 300 360 420 480
Time / min
Fig. 7. Time-course of nitrobenzene aromatic derivatives formed during anodic
oxidation of nitrobenzene aqueous solution on BDD anode: conditions: initialconcentration: 2 mM, current density: j = 60 mA cm2; electrolyte 0.05 M Na2SO4;
pH = 3 and at T = 20 °C.
Glyoxilic Acid
Oxalic Acid
Malonic Acid
Time / min
Fig. 8. Time-course of the concentration of relevant carboxylic acids detected
during anodic oxidation of nitrobenzene aqueous solution on BDD anode: condi-
tions: initial concentration: 2 mM, current density: j = 60 mA cm2; electrolyte0.05 M Na2SO4; pH = 3 and at T = 20 °C.
Fig. 10. Proposed reaction pathway for the mineralization of nitrobenzene in
aqueous acid medium by hydroxyl radicals generated in anodic oxidation process.
Concentration / mgL
3.4. Reaction sequence for nitrobenzene degradation
Time/ min
presents a plausible general pathway for the mineraliza-
Fig. 9. Accumulation of nitrate (j) and ammonium (N) ions detected during anodic
tion of nitrobenzene in acid medium by anodic oxidation. The
oxidation of nitrobenzene aqueous solution on BDD anode: conditions: initial
proposed sequence takes into account all products detected for
concentration: 2 mM, current density: j = 60 mA cm2; electrolyte 0.05 M Na2SO4;
nitrobenzene in this work and for sake of simplicity. This result
pH = 3 and at T = 20 °C.
is significant, because there are few data in literature showingmechanisms for nitrobenzene degradation by hydroxyl radicals at-tack in acid medium. Then, the presented mechanism can help todetail nitrobenzene degradation mechanisms by others AOP's at
Organic nitrogen of NB converted to mineral ions such as NHþ
similar conditions.
and NO during its mineralization by anodic treatment was fol-
lowed by ion chromatographic analyses. The concentrations ofNHþ and NO were 2.9 mg L1 and 0.8 mg L1, respectively, after
480 min of electrolysis (). This result proves the total transfor-mation of organic nitrogen into mineral ions. These results demon-
The electrochemical oxidation of nitrobenzene, using the anodic
strate clearly that the mineralization of NB by the process of anodic
oxidation process with BDD anode, has been deeply investigated.
oxidation goes through carboxylic acids. This fact is illustrated in
This electrode behaves as a hydroxyl radical generator under con-
the studies of Brillas et al. who applied this method on other organ-
trolled conditions as a result of the water decomposition on the
electrode surface. Being very reactive species and having a very
N. Rabaaoui et al. / Separation and Purification Technology 107 (2013) 318–323
high oxidation power, these radicals ensure the mineralization of
[12] S. Hammami, N. Bellakhal, N. Oturan, M.A. Oturan, M. Dachraoui, Degradation
nitrobenzene in aqueous medium. The operational system param-
of Acid Orange 7 by electrochemically generated OH radicals in acidic aqueousmedium using a boron-doped diamond or platinum anode: a mechanistic
eters were optimized. The main conclusions of this work can be
study, Chemosphere 73 (2008) 678–684.
summarized in the following points:
[13] J.M. Aquino, R.C. Rocha-Filho, N. Bocchi, S.R. Biaggio, Electrochemical
degradation of the Acid Blue 62 dye on a b-PbO2 anode assessed by theresponse surface methodology, J. Appl. Electrochem. 40 (2010) 1751–1757.
(1) BDD-anodic oxidation can be used successfully to remove
[14] L. Ciríaco, C. Anjo, J. Correia, M.J. Pacheco, A. Lopes, Electrochemical
almost all the COD and TOC of synthetic wastewaters which
degradation of ibuprofen on Ti/Pt/PbO2 and Si/BDD electrodes, Electrochim.
are polluted by nitrobenzene.
Acta 54 (2009) 1464–1472.
[15] B. Adams, M. Tian, A. Chen, Design and electrochemical study of SnO
(2) The nature of the supporting electrolyte has a great influ-
mixed oxide electrodes, Electrochim. Acta 54 (2009) 1491–1498.
ence on the rate and the efficiency of the electrochemical
[16] D. Gandini, E. Mahe, P.A. Michaud, W. Haenni, A. Perret, C. Comninellis,
oxidation of nitrobenzene. The treatment in the presence
Oxidation of carboxylic acids at boron-doped diamond electrodes forwastewater treatment, J. Appl. Electrochem. 30 (2000) 1345–1350.
of Na2SO4 or Na3PO4 appears to be more efficient in the
[17] S. Ammar, R. Abdelhedi, C. Flox, C. Arias, E. Brillas, Electrochemical degradation
COD removal than that in the presence of NaCl or KCl.
of the dye indigo carmine at boron-doped diamond anode for wastewaters
Accordingly, the mediated oxidation by the electro-gener-
remediation, Environ. Chem. Lett. 4 (2006) 229–233.
ated reagents from the anodic oxidation of supporting elec-
[18] X.Y. Li, Y.H. Cui, Y.J. Feng, Z.M. Xie, J.D. Gu, Reaction pathways and mechanisms
of the electrochemical degradation of phenol on different electrodes, Water
trolyte plays an important role in the efficiency of the
Res. 39 (2005) 1972–1981.
[19] B. Boye, E. Brillas, B. Marselli, P.A. Michaud, C. Comninellis, G. Farnia, G.
(3) During bulk electrolyses at high current density, our product
Sandon, Electrochemical incineration of chloromethylphenoxy herbicides inacid medium by anodic oxidation with boron-doped diamond electrode,
is directly combusted to CO2 due to a high local concentra-
Electrochim. Acta 51 (2006) 2872–2880.
tion on the anode surface of electrogenerated hydroxyl rad-
[20] M.J. Pacheco, V. Santos, L. Ciríaco, A. Lopes, Electrochemical degradation of
icals relative to nitrobenzene.
aromatic amines on BDD electrodes, J. Hazard. Mater. 186 (2011) 1033–1041.
[21] S. Hattori, M. Doi, E. Takahashi, T. Kurosu, M. Nara, S. Nakamatsu, Y. Nishiki, T.
(4) The decay kinetics of nitrobenzene follows a pseudo-first-
Furuta, M. Iida, Electrolytic decomposition of amarantha dyestuff using
order reaction. For NB degradation, phenol, catechol, resor-
diamond electrodes, J. Appl. Electrochem. 33 (2003) 85–91.
cinol, 1,2,4-trihydroxybenzene, hydroquinone and benzo-
[22] Y.J. Feng, X.Y. Li, Electro-catalytic oxidation of phenol on several metal-oxide
electrodes in aqueous solution, Water Res. 37 (2003) 2399–2407.
quinone are detected as aromatic intermediates which are
[23] J. Sun, H. Lu, H. Lin, L. Du, W. Huang, H. Li, Electrochemical oxidation of
oxidized as a mixture of short linear carboxylic acids.
aqueous phenol at low concentration using Ti/BDD electrode, Sep. Purif.
Technol. 88 (2012) 116–120.
[24] C. Comninellis, C. Pulgarin, Anodic oxidation of phenol for wastewater
treatment, J. Appl. Electrochem. 21 (1991) 703–708.
[25] M. Panizza, P.A. Michaud, G. Cerisola, C. Comninellis, Anodic oxidation of 2-
naphol diamond electrodes for wastewater treatment, J. Appl. Electrochem. 30
[1] F.D. Marvin-Sikkema, J.A.M. Bont, Degradation of nitroaromatic compounds by
(2000) 1345–1350.
microorganisms, Appl. Microbiol. Biotechnol. 42 (1994) 499–507.
[26] M. Panizza, G. Cerisola, Influence of anode material on the electrochemical
[2] J.C. Spain, Biodegradation of nitroaromatic compounds, Annu. Rev. Microbiol.
oxidation of 2-naphthol. Part 2. Bulk electrolysis experiments, Electrochim.
49 (1995) 523–555.
Acta 49 (2004) 3221–3226.
[3] V.L. Gemini, A. Gallego, V.M. de Oliveira, C.E. Gomez, G.P. Manfio, S.E. Korol,
[27] N. Rabaaoui, M.S. Allagui, Anodic oxidation of salicylic acid on BDD electrode:
variable effects and mechanisms of degradation, J. Hazard. Mater. 243 (2012)
Wratislaviensis, Int. Biodeterior. Biodegrad. 55 (2005) 103–108.
[4] V. Uberoi, S.K. Bhattacharya, Toxicity and degradability of nitrophenols in
[28] P. Cañizares, F. Larrondo, J. Lobato, M.A. Rodrigo, C. Sáez, Electrochemical
anaerobic systems, Water Environ. Res. 69 (1997) 146–156.
synthesis of peroxodiphosphate using boron-doped diamond anodes, J.
[5] M.C. Tomei, M.C. Annesini, S. Bussoletti, 4-Nitrophenol biodegradation in a
Electrochem. Soc. 152 (11) (2005) D191–D196.
sequencing batch reactor: kinetic study and effect of filling time, Water Res. 38
[29] P. Cañizares, B. Louhichi, A. Gadri, B. Nasr, R. Paz, M.A. Rodrigo, C. Saez,
(2004) 375–384.
[6] B.A. Donlon, E. Razo-Flares, G. Lettiga, J.A. Field, Continuous detoxification,
manufacturing process, J. Hazard. Mater. 146 (2007) 552–557.
transformation and degradation of nitrophenol in upflow anaerobic sludge
[30] X. Zhu, M. Tong, S. Shi, H. Zhao, J. Ni, Essential explanation of the strong
blanket (UASB) reactors, Biotechnol. Bioenergy 51 (1996) 439–449.
mineralization performance of boron-doped diamond electrodes, Environ. Sci.
[7] Z.I. Bhatti, H. Toda, K. Furukawa, P-Nitrophenol degradation by activated
Technol. 42 (2008) 4914–4920.
sludge attached on nonwovens, Water Res. 36 (2002) 1135–1142.
[31] C.A. Martinez-Huitle, A. De Battisti, S. Ferro, S. Reyna, M. Cerro-Lopez, M.A.
[8] M.C. Tomei, M.C. Annesini, R. Luberti, G. Cento, A. Senia, Kinetics of 4-
Quiroz, Removal of the pesticide methamidophos from aqueous solutions by
nitrophenol biodegradation in a sequencing batch reactor, Water Res. 37
electrooxidation using Pb/PbO2, Ti/SnO2, and Si/BDD electrodes, Environ. Sci.
(2003) 3803–3814.
Technol. 42 (2008) 6929–6935.
[9] P. Jiang, J. Zhou, A. Zhang, Y. Zhong, Electrochemical degradation of
[32] E. Weiss, K. Groenen-Serrano, A. Savall, A comparison of electrochemical
pnitrophenol with different processes, J. Environ. Sci. 22 (2010) 500–506.
degradation of phenol on boron-doped diamond and lead dioxide anodes, J.
[10] S. Yuan, M. Tian, Y. Cui, L. Lin, X. Lu, Treatment of nitrophenols by cathode
Appl. Electrochem. 38 (2008) 329–337.
reduction and electron-Fenton methods, J. Hazard. Mater. B137 (2006) 573–
[33] D.I. dos Santos, J.C. Afonso, A.B. Dutra, Electrooxidation of phenol on a Ti/RuO2
anode: effect of some electrolysis parameters, J. Braz. Chem. Soc. 22 (2011)
[11] W.B. Zhang, X.M. Xiao, T.C. An, Z.G. Song, J.M. Fu, G.Y. Sheng, M.C. Cui, Kinetics,
degradation pathway and reaction mechanism of advanced oxidation of 4-
[34] E. Brillas, S. Garcia-Segura, M. Skoumal, C. Arias, Electrochemical incineration
nitrophenol in water by a UV/H
of diclofenac in neutral aqueous medium by anodic oxidation using Pt and
2O2 process, J. Chem. Technol. Biotechnol. 78
(2003) 788–794.
boron-doped diamond anodes, Chemosphere 79 (2010) 605–612.
Source: http://www.ugaf.rnu.tn/Bibliotheque/Anodic%20oxidation%20of%20nitrobenzene%20on%20BDD%20electrode%20Variable%20effects.pdf
Occupational Safety and Health Information Series A GUIDE TO THE This booklet was written by Dr Chris Walls with assistance from theOSH Departmental Medical Practitioners and Dr Julian Crane(Physician, Wellington), Dr Margaret Wilsher (Physician, Auckland)and Dr Colin Wong (Physician, Dunedin), members of the NODSAsthma Panel. Dr John Allen (Pukekohe), Dr Charles Skinner (Auckland) and DrRob Stewart (Auckland) provided a critique from a general practiceview point and their assistance is greatly appreciated.
CATALOGO GENERALEGENERAL CATALOGUE made in Italy, made in F.A.R.G. Nei primi anni Sessanta ad Invorio, nella provincia di Novara, da sempre distretto di eccellenza nella produzione dell'industriadella rubinetteria, Giampiero Conton inizia la sua attività fondando la Rubinetteria Conton. Inizialmente l'azienda ebbe comescopo principale la commercializzazione di materiale idrosanitario; l'intuito del fondatore e alcuni segnali provenienti dallaclientela fecero capire le aperture del mercato e la possibilità di investire con ottimi risultati nella produzione di rubinetti agalleggiante con relative sfere in materiale plastico e in rame, senza dover fare i conti con una concorrenza troppo numerosa.E' nel 1996 che nasce F.A.R.G., naturale evoluzione di Rubinetteria Conton, che opera oggi su un'area di circa 15.000 mq dicui 5.000 mq coperti dedicati ai processi produttivi. Nel tempo la gamma dei prodotti si è ampliata con l'introduzione dialcuni componenti per impianti idrosanitari mantenendo la garanzia di qualità attestata da una produzione interamente ‘Made in Italy'. La costante attenzione della qualità, l'utilizzo di tecnologie avanzate e una rete di vendita che si avvale dellacollaborazione di agenti presenti sul territorio, hanno portato l'azienda a imporsi sul mercato nazionale e su quello estero.