Microsoft word - document7
1 According to the author of the module, the compulsory readings do not infringe known copyright.
COMPULSORY READINGS
Readings #1 and #2
List of relevant readings :FromTextbook Revolution-Taking the Bite out of Books
http://textbookrevolution.org/chemistry/
1. Virtual Textbook of Organic ChemistryOrganic Chemistry by Wil iam Reusch
2. Organic Chemistry by Richard and Sal y Daley
3.Organic Chemistry Practice Problems by Wil iam Reusch
Reading #3
Complete reference Aromaticity: http://en.wikipedia.org/wiki/Aromaticity 6th September 2006 also see the screen capture below: Aromaticity- Wikipedia, the free encyclopedia
Abstract :
A brief but important discussion on the concept of aromaticity.The discussion is
centred around the Kekulé model of the structure of benzene in which benzene is
considered a hybrid of two structures with alternating single and double bonds. The
article gives in a summary form the characteristics of aromatic compounds.
Rationale:
The article wil help you to study the major characteristics of aromatic compounds and prepare you better to attempt questions on aromaticity.
Additional Reading
Complete reference:
Benzene: From Wikipedia, the free encyclopedia
http://en.wikipedia.org/wiki/benzene 6th September 2006
SubstitutionReactions of benzene and other Aromatic compounds(see screen capture below
Abstract :
The article mostly deals with the structure of benzene from Kekulé's point of view that
it is aresonance stabilized structure whose bonds are neither ful y single nor double
but intermediate between the two, and that al bonds lengths in benzene are
equalent. The typical reactions of benzene are also highlighted in the article.
Rationale:
Although the article does not provide a comprehensive coverage of benzene and its
reactions, it t is enough to stimulate further reading for a more detailed understanding
of arenes and aromaticity.
Complete reference :From Wikipedia, the free encyclopedia
Pyrrole: http://en.wikipedia.org/wiki/pyrrole
Furan: http://en.wikipedia.org/wiki/furan
Thiophene: http://en.wikipedia.org/wiki/thiophene
Pyridine : http://en.wikipedia.org/wiki/pyridine
Abstract :The links above give free wikipedia articles on pyrrole, furan, thiophene
and pyridine, discussing their chemistry, physical properties, and uses. There are a
number of search engines that wil lead you to many other important topics on these
heterocyclic molecules. You are advised to fol ow these links to read more on the
chemistry of these important heterocyclics
Rationale:
Pyrole, furan and thiophene are three important aromatic heterocyclics that are found
in a number of natural products. These are aromatic systems that have reactions
similar to benzene in that they undergo substitution reactions. It is therefore important
that you learn of their chemistry. You wil realize that furan is more reactive than
benzene in that it even undergoes Diels-Alder reaction. Going throug these articles
you wil learn quite a good amount of chemistry of other heterocyclic compounds and
also fol ow other suggested web links.
Benzene and Other Aromatic Compounds
The adjective "aromatic" is used by organic chemists in a rather different way than it is normally applied. It has its origin in the observation that certain natural substances, such as cinnamon bark, wintergreen leaves, vanilla beans and anise seeds, contained fragrant compounds having common but unexpected properties. Cinnamon bark, for example, yielded a pleasant smelling compound, formula C9H8O, named cinnamaldehyde. Because of the low hydrogen to carbon ratio in this and other aromatic compounds (note that the H:C ratio in an alkane is >2), chemists expected their structural formulas would contain a large number of double or triple bonds. Since double bonds are easily cleaved by oxidative reagents such as potassium permanganate or ozone, and rapidly add bromine and chlorine, these reactions were applied to these aromatic compounds. Surprisingly, products that appeared to retain many of the double bonds were obtained, and these compounds exhibited a high degree of chemical stability compared with known alkenes and cycloalkenes (aliphatic compounds). On treatment with hot permanganate solution, cinnamaldehyde gave a stable, crystalline C7H6O2 compound, now called benzoic acid. The H:C ratio in benzoic acid is <1, again suggesting thepresence of several double bonds. Benzoic acid was eventually converted to the stable hydrocarbon benzene, C6H6, which also proved unreacive to common double bond transformations, as shown below. For comparison, reactions of cyclohexene, a typical alkene, with these reagents are also shown (green box). As experimental evidence for a wide assortment of compounds was acquired, those incorporating this exceptionally stable six-carbon core came to be called "aromatic".
If benzene is forced to react by increasing the temperature and/or by addition of a catalyst, It
undergoes
substitution reactions rather than the addition reactions that are typical of alkenes. This
further confirms the previous indication that the six-carbon benzene core is unusually stable to
chemical modification. The conceptual contradiction presented by a high degree of unsaturation (low
H:C ratio) and high chemical stability for benzene and related compounds remained an unsolved
puzzle for many years. Eventually, the presently accepted structure of a regular-hexagonal, planar
ring of carbons was adopted, and the exceptional thermodynamic and chemical stability of this system
was attributed to resonance stabilization of a conjugated cyclic triene.
Benzene:
Here, two structurally and energetically equivalent electronic structures for a stable compound are written, but no single structure provides an accurate or even an adequate representation of the true molecule. The six-membered ring in benzene is a perfect hexagon (all carbon-carbon bonds have an identical length of 1.40 Å). The cyclohexatriene contributors would be expected to show alternatingbond lengths, the double bonds being shorter (1.34 Å) than the single bonds (1.54 Å). An alternativerepresentation for benzene (circle within a hexagon) emphasizes the pi-electron delocalization in this molecule, and has the advantage of being a single diagram. In cases such as these, the electron delocalization described by resonance enhances the stability of the molecules, and compounds composed of such molecules often show exceptional stability and related properties.
Evidence for the enhanced thermodynamic stability of benzene was obtained from measurements of
the heat released when double bonds in a six-carbon ring are hydrogenated (hydrogen is added
catalytically) to give cyclohexane as a common product. In the following diagram cyclohexane
represents a low-energy reference point. Addition of hydrogen to cyclohexene produces cyclohexane
and releases heat amounting to 28.6 kcal per mole. If we take this value to represent the energy cost
of introducing one double bond into a six-carbon ring, we would expect a cyclohexadiene to release
57.2 kcal per mole on complete hydrogenation, and 1,3,5-cyclohexatriene to release 85.8 kcal per
mole. These
heats of hydrogenation would reflect the relative thermodynamic stability of the
compounds. In practice, 1,3-cyclohexadiene is slightly more stable than expected, by about 2 kcal,
presumably due to conjugation of the double bonds.
Benzene, however, is an extraordinary 36
kcal/mole more stable than expected. This sort of stability enhancement is now accepted as a
characteristic of all aromatic compounds.
A molecular orbital description of benzene provides a more satisfying and more general treatment of "aromaticity". We know that benzene has a planar hexagonal structure in which all the carbon atoms are sp2 hybridized, and all the carbon-carbon bonds are equal in length. As shown below, theremaining cyclic array of six p-orbitals ( one on each carbon) overlap to generate six molecularorbitals, three bonding and three antibonding. The plus and minus signs shown in the diagram do not
represent electrostatic charge, but refer to phase signs in the equations that describe these orbitals
(in the diagram the phases are also color coded). When the phases correspond, the orbitals overlap
to generate a common region of like phase, with those orbitals having the greatest overlap (e.g. π 1)
being lowest in energy. The remaining carbon valence electrons then occupy these molecular orbitals
in pairs, resulting in a fully occupied (6 electrons) set of bonding molecular orbitals. It is this completely
filled set of bonding orbitals, or
closed shell, that gives the benzene ring its thermodynamic and
chemical stability, just as a filled valence shell octet confers stability on the inert gases.
The Molecular Orbitals of Benzene
To see an animated model of the benzene pi-molecular orbitals Click Here
To examine a Chime model of the p-orbital components of these benzene pi-orbitals.
Fused Benzene Ring Compounds
Benzene rings may be joined together (fused) to give larger polycyclic aromatic compounds. A few examples are drawn below, together with the approved numbering scheme for substituted derivatives. The peripheral carbon atoms (numbered in all but the last three examples) are all bonded to hydrogen atoms. The six benzene rings in coronene are fused in a planar ring; whereas the six rings in hexahelicene are not joined in a larger ring, but assume a helical turn, due to the crowding together of the terminal ring atoms. This helical configuration renders the hexihelicene molecule chiral, and it has been resolved into stable enantiomers having specific rotations of 3700º. Chime models of corannulene, coronene and hexahelicene may be examined by clicking on the appropriate structure in
the diagram.
As these extended aromatic compounds become larger, the ratio of hydrogen to carbon decreases.
For example, the symmetrical hexacyclic compound coronene has a H/C ratio =1/2, compared with 1
for benzene. If we were to imagine fused ring systems of this kind to be further extended in space, the
H/C ratio would approach zero, and the resulting compound would be a form of carbon. Such a carbon
allotrope exists and is called
graphite. Another well-characterised carbon allotrope is
diamond. The
structures for these two forms of carbon are very different, and are displayed below. Diamond is an
extended array of sp3 hybridized carbon atoms; whereas, graphite consists of overlapping sheets of
sp2 hybridized carbon atoms arranged in a hexagonal pattern. You may examine Chime models of
partial diamond and graphite structures by clicking on the appropriate structure below.
A comparison of the coronene and corannulene models discloses an interesting difference in their shapes. Coronene is absolutely flat and, aside from the peripheral hydrogens, resembles a layer of graphite. Its very high melting point reflects this resemblance. Corannulene, on the other hand, is slightly curved, resulting in a bowl-like shape.
If we extend the structure of corannulene by adding similar cycles of
five benzene rings, the curvature of the resulting molecule should
increase, and eventually close into a sphere of carbon atoms. The
archtypical compound of this kind (C60) has been named
buckminsterfullerene because of its resemblance to the geodesic
structures created by Buckminster Fuller. It is a member of a family of
similar carbon structures that are called
fullerenes. These materials
represent a third class of carbon allotropes. Alternating views of the
C60 fullerene structure are shown on the right, together with a soccer
ball-like representation of the 12 five and 20 six-membered rings
composing its surface. By clicking on this graphic, a Chime model of C60 will be displayed.
Although C60 is composed of fused benzene rings its chemical reactivity resembles that of the
cycloalkenes more than benzene. Indeed, exposure to light and oxygen slowly degrade fullerenes to
cage opened products. Most of the reactions thus far reported for C60 involve addition to, rather than
substitution of, the core structure. These reactions include hydrogenation, bromination and
hydroxylation. Strain introduced by the curvature of the surface may be responsible for the enhanced
reactivity of C60.
. Larger fullerenes, such as C70, C76, C82 & C84have elipsoidal or distorted spherical structures, and
fullerene-like assemblies up to C240 have been detected. A fascinating aspect of these structures is
that the space within the carbon cage may hold atoms, ions or small molecules. Such species are
called
endohedral fullerenes. The cavity of C60 is relatively small, but encapsulated helium, lithium
and atomic nitrogen compounds have been observed. Larger fullerenes are found to encapsulate
lanthanide metal atoms.
Interest in the fullerenes has led to the discovery of a related group of carbon structures referred to as nanotubes. As shown in the following illustration, nanotubes may be viewed as rolled up segments of graphite. The chief structural components are six-membered rings, but changes in tube diameter, branching into side tubes and the capping of tube ends is accomplished by fusion with five and seven-membered rings. Many interesting applications of these unusual structures have been proposed.
Other Aromatic Systems
Many unsaturated cyclic compounds have exceptional properties that we now consider characteristic of "aromatic" systems. The following cases are illustrative:
Addition ( 0 ºC )
Addition ( 0 ºC )
Addition ( 0 ºC )
Substitution ( 0 ºC )
The first three compounds (cyclic polyenes) have properties associated with alkenes in general. Eachreacts readily with bromine to give addition products, as do most alkenes. The thermodynamic changeon introducing double bonds into the carbon atom ring is also typical of alkenes (a destabilization of ca. 26 kcal/mol for each double bond). Conjugation offsets this increase in energy by a small amount (4-6kcal/mol).
The remaining four compounds exhibit very different properties, and are considered aromatic.
Benzene and pyridine are relatively unreactive with bromine, requiring heat and/or catalysts to force
reaction, the result of which is substitution rather than addition. Furan and pyrrole react more rapidly
with bromine, but they also give substitution products. This tendency to favor substitution rather than
addition suggests that the parent unsaturated ring system has exceptional stability. Thermodynamic
measurements support this conclusion. The enhanced stability, often referred to as
aromatic
stabilization, ranges (in the above cases) from a low of 16 kcal/mol for furan to 36 kcal/mol for
benzene.
Factors Required for Aromaticity
A planar (or near planar) cycle of sp2 hybridized atoms, the p-orbitals of which are orientedparallel to each other. These overlapping p-orbitals generate an array of π-molecular orbitals.
These π-orbitals are occupied by 4n+2 electrons (where n is an integer or zero). This
requirement is known as
The Hückel Rule. All the aromatic compounds discussed above have 6
π-electrons (n=1).
1,3-Cyclopentadiene and 1,3,5-cycloheptatriene both fail to meet the first requirement, since one
carbon atom of each ring is sp3 hybridized and has no p-orbital. Cyclooctatetraene fails bothrequirements, although it has a ring of sp2 hybridized atoms. This molecule is not planar ( a geometrythat would have 135º bond angles ). Angle strain is relieved by adopting a tub-shaped conformation;consequently, the p-orbitals can only overlap as isolated pairs, not over the entire ring. Furthermore,cyclooctatetraene has 8 π-electrons, a number not consistent with the Hückel Rule.
Benzene is the archetypical aromatic compound. It is planar, bond angles=120º, all carbon atoms inthe ring are sp2 hybridized, and the pi-orbitals are occupied by 6 electrons. The aromatic heterocyclepyridine is similar to benzene, and is often used as a weak base for scavanging protons. Furan and pyrrole have heterocyclic five-membered rings, in which the heteroatom has at least one pair of non-bonding valence shell electrons. By hybridizing this heteroatom to a sp2 state, a p-orbitaloccupied by a pair of electrons and oriented parallel to the carbon p-orbitals is created. The resultingplanar ring meets the first requirement for aromaticity, and the π-system is occupied by 6 electrons,4 from the two double bonds and 2 from the heteroatom, thus satisfying the Hückel Rule.
Four illustrative examples of aromatic compounds are shown above. The sp2 hybridized ring atomsare connected by brown bonds, the π-electron pairs and bonds that constitute the aromatic ring arecolored blue. Electron pairs that are not part of the aromatic π-electron system are black. The firstexample is azulene, a blue-colored 10 π-electron aromatic hydrocarbon isomeric with naphthalene.
The second and third compounds are heterocycles having aromatic properties. Pyridine has abenzene-like six-membered ring incorporating one nitrogen atom. The non-bonding electron pair onthe nitrogen is not part of the aromatic π-electron sextet, and may bond to a proton or otherelectrophile without disrupting the aromatic system. In the case of thiophene, a sulfur analog of furan,one of the sulfur electron pairs (colored blue) participates in the aromatic ring π-electron conjugation.
The last compound is imidazole, a heterocycle having two nitrogen atoms. Note that only one of thenitrogen non-bonding electron pairs is used for the aromatic π-electron sextet. The other electronpair (colored black) behaves similarly to the electron pair in pyridine.
Monocyclic compounds made up of alternating conjugated double bonds are called
annulenes.
Benzene and 1,3,5,7-cyclooctatetraene are examples of annulenes; they are named [6]annulene and
[8]annulene respectively, according to a general nomenclature system in which the number of
pi-electrons in an annulene is designated by a number in brackets. Some annulenes are aromatic (e.g.
benzene), but many are not due to non-planarity or a failure to satisfy the Hückel Rule. Compounds
classified as [10]annulenes (a Hückel Rule system) serve to illustrate these factors.
As shown in the following diagram, 1,3,5,7,9-cyclodecapentaene fails to adopt a planar conformation,
either in the all cis-configuration or in its 1,5-trans-isomeric form. The transannular hydrogen crowding
that destabilizes the latter may be eliminated by replacing the interior hydrogens with a bond or a short
bridge (colored magenta in the diagram). As expected, the resulting 10 π-electron annulene
derivatives exhibit aromatic stability and reactivity as well as characteristic ring current anisotropy in
the nmr. Naphthalene and azulene are [10]annulene analogs stabilized by a transannular bond. Although the CH2 bridged structure to the right of naphthalene in the diagram is not exactly planar, theconjugated 10 π-electron ring is sufficiently close to planarity to achieve aromatic stabilization. Thebridged [14]annulene compound on the far right, also has aromatic properties.
A modified [10]annulene, aromatic by nmr criteria, was prepared recently by chemists at CaliforniaInstitute of Technology. Remarkably, this hydrocarbon is chemically unstable, in contrast to most other aromatic hydrocarbons. To learn more Click Here.
Carbanions and carbocations may also show aromatic stabilization. Some examples are:
The three-membered ring cation has 2 π-electrons and is surprisingly stable, considering its ringstrain. Cyclopentadiene is as acidic as ethanol, reflecting the stability of its 6 π-electron conjugatebase. Salts of cycloheptatrienyl cation (tropylium ion) are stable in water solution, again reflecting thestability of this 6 π-electron cation.
Conjugated ring systems having 4n π-electrons (e.g. 4, 8, 12 etc. electrons) not only fail to show anyaromatic properties, but appear to be less stable and more reactive than expected. As noted above,1,3,5,7-cyclooctatetraene is non-planar and adopts a tub-shaped conformation. The compound isreadily prepared, and undergoes addition reactions typical of alkenes. Catalytic hydrogenation of thistetraene produces cyclooctane. Planar bridged annulenes having 4n π-electrons have proven to berelatively unstable. Examples of 8 and 12-π-electron systems are shown below, together with a
similar 10 π-electron aromatic compound.
The simple C8H6 hydrocarbon pentalene does not exist as a stable compound, and its hexaphenyl
derivative is air sensitive. The 12-π-electron analog heptalene has been prepared, but is also
extremely reactive (more so than cyclooctatetraene). On the other hand, azulene is a stable
10-π-electron hydrocarbon that incorporates structural features of both pentalene and heptalene.
Azuleneis a stable blue crystalline solid that undergoes a number of typical aromatic substitution
reactions. The unexpected instability of 4n π-electron annulenes has been termed "
antiaromaticity".
Other examples may be cited. Thus, all attempts to isolate 1,3-cyclobutadiene have yielded its dimer,
or products from reactions with other compounds introduced into the reaction system. Similarly,
cyclopentadienyl cation (4 π-electrons) and cycloheptatrienyl anion (8 π-electrons) show very high
reactivity when forced to form.
Cyclooctatetraene is a fascinating compound. To see more of its
chemistry Click Here.
Return to Table of Contents
Nucleophilicity & Basicity
Nucleophilicity and Basicity Factors in Organic
Recall the definitions of electrophile and nucleophile:
Electrophile: An electron deficient atom, ion or molecule that has an affinity for an electron pair, and will bond to a base or nucleophile.
Nucleophile: An atom, ion or molecule that has an electron pair that may be donated in bonding to an electrophile (or Lewis acid).
Definitions and examples of acids and bases were provided earlier in this text, and should be reviewed if the reader is not comfortable with this subject.
Many functional groups have weakly electrophilic carbon atoms (colored red in the following
examples). These include
alkyl halides and
sulfonate esters {
C-X and
C-OSO2R}, as well as carbonyl
compounds such as
aldehydes and
ketones {
C=O}. These electrophilic functions may react with
nucleophiles (bases) in two distinct ways:
(i) Substitution or addition at carbon (this reflects nucleophilicity)
(ii) β-Elimination or enolate anion formation (this reflects basicity)
Because these electrophilic reactants are weak, such reactions normally require strong nucleophiles
or bases to proceed. However, if a preliminary ionization to a strongly electrophilic carbocation
occurs: [ C-X ——
> C(+) + X(–) ]
or if the carbonyl group is converted to its more electrophilic conjugate acid: [ C=O + A(+) ——
>
(+)C-O-A]
then reactions with much weaker nucleophiles or bases may take place.
Some confusion in distinguishing basicity (base strength) and nucleophilicity (nucleophile strength) is
inevitable. Since basicity is a less troublesome concept; it is convenient to start with it.
Basicity refers to the ability of a base to accept a
proton. Basicity may be related to the pKa of the
corresponding conjugate acid, as shown below. The strongest bases have the weakest conjugate
acids and
vice versa. The range of basicities included in the following table is remarkable, covering
over fifty powers of ten!
In an acid-base equilibrium the weakest acid and the weakest base will predominate (they will
necessarily be on the same side of the equilibrium). Learning the pKa values for common compounds
provides a useful foundation on which to build an understanding of acid-base factors in reaction
mechanisms.
RS(–) CN(–) RO(–) NH2
Conj. Acid HI
HCl H3O(+) CH3CO2H RSH HCN ROH NH3
Nucleophilicity is a more complex property. It commonly refers to the rate of substitution reactions at
the halogen-bearing
carbon atom of a reference alkyl halide, such as CH3-Br. Thus the
nucleophilicity of the Nu:(–) reactant in the following substitution reaction varies as shown in the chart
below:
3CO2 (–) < Cl(–) < Br(–) < N3
< CH3O(–) < CN(–) < I(–) < CH3S(–)
The reactivity range encompassed by these reagents is over 5,000 fold, thiolate being the most reactive. Clearly, there are significant differences between these nucleophilicities and the basicitiesdiscussed above.
Some useful trends have been documented: (i) For a given element, negatively charged species are more nucleophilic (and basic) than are equivalent neutral species.
(ii) For a given period of the periodic table, nucleophilicity (and basicity) decreases on moving from left to right.
(iii) For a given group of the periodic table, nucleophilicity increases from top to bottom (
i.e. with increasing size),
although there is a solvent dependence due to hydrogen bonding.
Basicity varies in the opposite manner.
For two or more molecules incorporating nucleophilic atoms of the same kind and charge, the stronger
base is usually the stronger nucleophile. Thus, 2,2,2-trifluroethoxide (pKa 12) is a weaker base and
nucleophile than ethoxide (pKa 16). A notable exception to this rule occurs when a vicinal (adjacent)
atom carries a non-bonding electron pair. Two common examples of this exception, called
the alpha
effect, are hydroxide ion (pKa 15.7) compared with hydroperoxide ion (pKa 11.6), and ammonia (pKa
9.3) compared with hydrazine (pKa 8.0). In each of these pairs the weaker base is the stronger
nucleophile.
Solvation of nucleophilic anions markedly influences their reactivity. The
nucleophilicities cited above were obtained from reactions in methanol solution.
Polar, protic solvents such as water and alcohols solvate anions by hydrogen
bonding interactions, as shown in the diagram on the right. These solvated
species are more stable and less reactive than the unsolvated "naked" anions. Polar, aprotic solvents such as DMSO (dimethyl sulfoxide), DMF (dimethylformamide) and acetonitrile do not solvate anions nearly as well as methanol, but provide good solvation of the accompanying cations. Consequently, most of the nucleophiles discussed here react more rapidly in solutions prepared from these solvents. These solvent effects are more pronounced for small basic anions than for large weakly basic anions. Thus, for reaction in DMSO solution we observe the following reactivity order:
Nucleophilicity: I(–) < Br(–) < Cl(–) N (–)
< CH3CO2 (–) < CN(–) CH3S(–) < CH3O(–)
Note that this order is roughly the order of increasing basicity (see above).
As we have noted, many common organic reactions proceed by bonding between nucleophilic andelectrophilic sites in the reactant molecules. Three examples are shown in equations 1 through 3;electrophiles are colored red, and nucleophiles are colored blue.
Reaction #1 is an example of a SN2 substitution reaction. The electrophilic carbon of 1-chlorobutane isattacked by the nucleophilic bromide anion in a single-step displacement process. The curly bracketsenclose a structure for the transition state in this reaction.
Reactions #2 and #3 are two-step sequences. In the former addition reaction, bromine (anelectrophile) attacks the nucleophilic double bond of 1-butene to give an electrophilic cyclic-bromoniumintermediate (enclosed in square brackets) accompanied by a nucleophilic bromide ion. This ion-pairis very short-lived, another nucleophile-electrophile bonding reaction leads to the product(1,2-dibromobutane). In reaction #3 (a substitution reaction) the electrophilic carbonyl carbon atombonds to the nucleophilic oxygen atom of ethyl alcohol to give an intermediate (in square brackets)
that eliminates HCl, yielding the ester ethyl acetate. In all of these examples the reactivity of theelectrophiles and nucleophiles is sufficient to allow reaction to proceed spontaneously at roomtemperature.
It is not difficult, however, to find very similar combinations of compounds that either fail to react at all, or do so extremely slowly. Equations 4 through 6 illustrate this behavior for analogs of the first threereactions.
The factors that influence such differences in reactivity may be complex, but in the above cases arelargely due to a poor anionic leaving group (eq. 4); aromatic stabilization and reduced nucleophilicity ofa conjugated π-electron system (eq. 5); and reduced electrophilic character of a substituted carbonylgroup (eq. 6).
First, compare reaction #4 with #1. Since oxygen is slightly more electronegative than chlorine (3.5 vs.
2.8 on the Pauling scale), we might expect a C-O bond to be more polar than a C-Cl bond. A bettermeasure of the electrophilic character of a carbon atom in a compound comes from nmr chemicalshifts (both 1H & 13C), and these indicate that oxygen and chlorine substituents exert similar effectswhen bonded to sp3 hybridized carbon atoms. In any event, the failure of reaction #4 cannot be due todifferences in the electrophilicity and nucleophilicity of the reactants.
The key factor here is the stability of the leaving anion (chloride vs. hydroxide). We know that HCl is amuch stronger acid than water (by more than 15 powers of ten), and this difference will be reflected inreactions that generate their conjugate bases. Thus, chloride anion is much more stable and lessreactive than is hydroxide anion, so the former is a better and more common leaving group. Indeed,the failure of alcohols to undergo SN2 substitution reactions makes them useful solvents for manysuch reactions, including #1.
In the case of reaction #5, the aromatic stabilization of the benzene ring makes it less susceptible toattack by electrophiles than are simple alkenes. Thus, elemental bromine is not sufficientlyelectrophilic to induce a reaction with benzene, even though the latter is nucleophilic.
Lastly, reactions #3 and #6 illustrate differences in the reactivity of carbonyl compounds. We knowthat the carbon atom of a carbonyl group is electrophilic and undergoes reaction with a variety ofnucleophiles. However, this electrophilic character may be enhanced or diminished by substituents. Ifwe take saturated aldehydes (RCH=O) as a reference, the additional alkyl substituent present inketones slightly reduces this electrophilicity, but the general reactivity pattern of these classes issimilar. On the other hand, a chlorine substituent is inductively electron withdrawing and increases the
electrophilicity of the carbonyl carbon significantly. Thus, acid chlorides are very reactive with a widerange of nucleophiles, including water and alcohols (eq. 3).
The oxygen and nitrogen substituents present in esters and amides have a similar inductive effect, butalso a pronounced electron donating character through a resonance interaction. Consequently, thecarbonyl carbon atom becomes less electrophilic, and these functional groups are less reactive than other carbonyl compounds. Thus, the failure of ethyl acetate to react with water (eq. 6) reflects thereduced electrophilic character of its carbonyl group.
Fortunately, these retarding factors can often be overcome by acid or base catalysis, which in general enhances electrophilicity (acids) or nucleophilicity (bases). Equations 7 through 9 show how this tacticmay be effectively applied to the unreactive examples given above.
In strong acid, reaction #4 proceeds nicely, as shown in equation 7. This is because the leaving group
has changed from hydroxide anion to water (the acidity of the conjugate acid H3O(+) is nearly that of
HCl).
In the second example, there are two obvious ways of circumventing the failure of benzene to react
with bromine:
• The bromine can be made more electrophilic
• The benzene ring can be made more nucleophilic.
The first tactic can be implemented by mixing bromine with ferric bromide, a Lewis acid catalyst. This
generates the bromonium cation, Br(+), a powerful electrophile. Equation #8 illustrates this approach.
The second tactic requires that the benzene ring be activated (made more nucleophilic) by
substitution with an electron donating group such as OH or NH2. For example, we find that phenol
(hydroxybenzene) reacts rapidly with bromine in the absence of any catalyst.
Finally, we can see that there are two ways of facilitating the ester hydrolysis reaction.:
•We can use a stronger nucleophile than water, such as hydroxide anion.
•We can increase the electrophile reactivity by converting the ester to its conjugate acid,
CH3C(OH)OC2H5 (+).
Equation #9 shows the former approach, which is an example of base catalysis. Acid catalysis of thereaction also works well.
Return to Table of Contents
Aromaticity - Wikipedia, the free encyclopedia
From Wikipedia, the free encyclopedia
This article is about a chemical property of molecules. For meanings related to odor, see aromacompound.
Aromaticity is a chemical property in which a conjugated
ring of unsaturated bonds, lone pairs, or empty orbitals
exhibit a stabilization stronger than would be expected by
the stabilization of conjugation alone. It can also be
considered a manifestation of cyclic delocalization and of
resonance [1] [2] [3].
This is usually considered to be because electrons are freeto cycle around circular arrangements of atoms, which arealternately single- and double-bonded to one another. These bonds may be seen as a hybrid of a single bondand a double bond, each bond in the ring identical to every other. This commonly-seen model of aromaticrings was developed by Kekulé. The model for benzene consists of two resonance forms, which correspondsto the double and single bonds' switching positions. Benzene is a more stable molecule than would beexpected of the theoretical molecule
cyclohexatriene.
1 Theory2 History3 Characteristics of aromatic compounds4 Aromatic compound classifications
4.1 Heterocyclics4.2 Polycyclics4.3 Substituted aromatics
5 Aromaticity in other systems6 See also7 References
By convention, the double-headed arrow indicates that the two structures are simplyhypothetical, since neither is an accurate representation of the actual compound. Theactual molecule is best represented by a hybrid (average) of these structures, which canbe seen at right. A C=C bond is shorter than a C-C bond, but benzene is perfectlyhexagonal--all six carbon-carbon bonds have the same length, intermediate between thatof a single and that of a double bond.
A better representation is that of the circular π bond (Armstrong's
inner cycle), in whichthe electron density is evenly distributed through a π bond above and below the ring. This model morecorrectly represents the location of electron density within the aromatic ring.
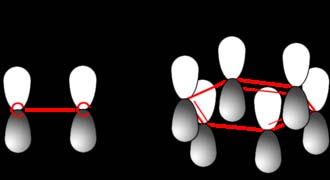
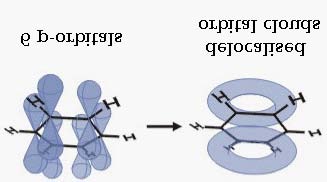
Aromaticity - Wikipedia, the free encyclopedia
The single bonds are formed with electrons in line between the carbon nuclei--these are called sigma bonds.
Double bonds consist of a sigma bond and another bond--a π bond. The π-bonds are formed from overlapof atomic p-orbitals above and below the plane of the ring. The following diagram shows the positions ofthese p-orbitals:
Since they are out of the plane of the atoms, these orbitals can interact with each other freely, and becomedelocalised. This means that instead of being tied to one atom of carbon, each electron is shared by all six inthe ring. Thus, there are not enough electrons to form double bonds on all the carbon atoms, but the "extra"electrons strengthen all of the bonds on the ring equally. The resulting molecular orbital has π symmetry.
The attribution of this exceptional stability is conventionally to Sir Robert Robinson,who was apparently the first (in 1925) [4] to coin the term aromatic sextet as a groupof six electrons that resists disruption.
In fact, this concept can be traced further back, via Ernest Crocker in 1922, [5] to
Henry Edward Armstrong, who in 1890, in an article entitled The structure of cycloid
hydrocarbons, wrote the (six) centric affinities act within a cycle.benzene may be
represented by a double ring (sic) . and when an additive compound is formed, the
inner cycle of affinity suffers disruption, the contiguous carbon-atoms to which
nothing has been attached of necessity acquire the ethylenic condition [6].
Here he is describing at least four modern concepts. Firstly his affinity is better known nowadays as the
electron, which was only to be discovered seven years later by J. J. Thomson. Secondly, he is describing
electrophilic aromatic substitution proceeding (thirdly) through a Wheland intermediate, in which (fourthly)
the conjugation of the ring is broken. He introduced the symbol C centered on the ring as a shorthand for
Aromaticity - Wikipedia, the free encyclopedia
the inner cycle, thus anticipating Eric Clar's notation. Arguably, he also anticipated the nature of wavemechanics, since he recognized that his affinities had direction, not merely being point particles, andcollectively having a distribution that could be altered by introducing substituents onto the benzene ring(much as the distribution of the electric charge in a body is altered by bringing it near to another body). Thequantum mechanical origins of this stability, or aromaticity, were first modelled by Hückel in 1931.
Characteristics of aromatic compounds
An aromatic compound contains a set of covalently-bound atoms with specific characteristics:
1. A delocalized conjugated π system, most commonly an arrangement of alternating single and double
2. Coplanar structure, with all the contributing atoms in the same plane3. Contributing atoms arranged in one or more rings4. A number of π delocalized electrons that is even, but not a multiple of 4. This is known as Hückel's
rule. Permissible numbers of π electrons include 2, 6, 10, 14, and so on
5. Special reactivity in organic reactions such as electrophilic aromatic substitution and nucleophilic
aromatic substitution
Whereas benzene is aromatic (6 electrons, from 3 double bonds), cyclobutadiene is not, since the number of
π delocalized electron is 4, which of course is a multiple of 4. The cyclobutadienide (2-) ion, however, isaromatic (6 electrons). An atom in an aromatic system can have other electrons that are not part of thesystem, and are therefore ignored for the 4n + 2 rule. In furan, the oxygen atom is sp2 hybridized. One lonepair is in the π system and the other in the plane of the ring (analogous to C-H bond on the other positions).
There are 6 π electrons, so furan is aromatic.
Aromatic molecules typically display enhanced chemical stability, compared to similar non-aromaticmolecules. The circulating π electrons in an aromatic molecule generate significant local magnetic fields thatcan be detected by NMR techniques. NMR experiments show that protons on the aromatic ring are shiftedsubstantially further down-field than those on aliphatic carbons. Planar monocyclic molecules containing 4n
π electrons are called antiaromatic and are, in general, destabilized. Molecules that could be antiaromaticwill tend to alter their electronic or conformational structure to avoid this situation, thereby becomingnon-aromatic. For example, cyclooctatetraene (COT) distorts itself out of planarity, breaking π overlapbetween adjacent double bonds. Möbius aromaticity describes a special case of aromaticity.
Aromatic molecules are able to interact with each other in so-called π-π stacking: the π systems form twoparallel rings overlap in a "face-to-face" orientation. Aromatic molecules are also able to interact with eachother in an "edge-to-face" orientation: the slight positive charge of the substituents on the ring atoms of onemolecule are attracted to the slight negative charge of the aromatic system on another molecule.
Many of the earliest-known examples of aromatic compounds, such as benzene and toluene, have distinctivepleasant smells. This property led to the term "aromatic" for this class of compounds, and hence to"aromaticity" being the eventually-discovered electronic property of them.
Aromatic compound classifications
The key aromatic hydrocarbons of commercial interest are benzene, toluene, ortho-xylene and para-xylene.
About 35 million tonnes are produced worldwide every year. They are extracted from complex mixturesobtained by the refining of oil or by distillation of coal tar, and are used to produce a range of importantchemicals and polymers, including styrene, phenol, aniline, polyester and nylon.
Aromaticity - Wikipedia, the free encyclopedia
In heterocyclic aromatics, one or more of the atoms in the aromatic ring is of an element other than carbon.
Pyridine is used as a solvent and chemical intermediate. Furan is aromatic, but not as aromatic as benzene,and therefore is more reactive. Furan is used as a chemical intermediate. Furan is also a carcinogen. Otherexamples include imidazole, pyrazole, oxazole, thiophene, and their benzannulated analogs (benzimidazole,for example).
Polycyclic aromatic hydrocarbons (PAH) are molecules containing two or more simple aromatic rings fusedtogether by sharing two neighboring carbon atoms (see also simple aromatic rings). Examples arenaphthalene, anthracene and phenanthrene.
Many chemical compounds contain simple aromatic rings in their structure. Examples are DNA whichcontains purine and pyrimidine, trinitrotoluene (TNT), acetylsalicylic acid (aspirin) and paracetamol.
Aromaticity in other systems
Aromaticity is found in ions as well: the cyclopropenyl cation (2e system), the cyclopentadienyl anion (4e),the tropylium ion (6e) and the cyclooctatetraene dianion (10e). Aromatic properties have been attributed tonon-benzenoid compounds such as tropone. Aromatic properties are tested to the limit in a class ofcompounds called cyclophanes.
A special case of aromaticity is found in homoaromaticity where conjugation is interrupted by a single sp3hybridized carbon atom. When carbon in benzene is replaced by other elements in borabenzene,silabenzene, germanabenzene, stannabenzene, phosphorine or pyrylium salts the aromaticity is still retained.
Aromaticity is also not limited to compounds of carbon, oxygen and nitrogen.
Metal aromaticity is believed to exist in certain metal clusters of aluminium. Möbius aromaticity occurs whena cyclic system of molecular orbitals formed from pπ atomic orbitals and populated in a closed shell by 4n(n is an integer) electrons is given a single half-twist to correspond to a Möbius topology. Because the twistcan be left-handed or right-handed, the resulting Möbius aromatics are dissymmetric or chiral. Aromaticswith two half-twists corresponding to the paradromic topologies first suggested by Johann Listing have beenproposed by Rzepa in 2005 [7]. In carbo-benzene the ring bonds are extended with alkyne and allenegroups.
Aromatic hydrocarbonsAromatic aminesPAHSimple aromatic ring
1. P. v. R. Schleyer, "Aromaticity (Editorial)", Chemical Reviews, 2001, 101, 1115-1118. DOI:
10.1021/cr0103221 Abstract (http://dx.doi.org/10.1021/cr0103221) .
Aromaticity - Wikipedia, the free encyclopedia
2. A. T. Balaban, P. v. R. Schleyer and H. S. Rzepa, "Crocker, Not Armit and Robinson, Begat the Six
Aromatic Electrons", Chemical Reviews, 2005, 105, 3436-3447. DOI: 10.1021/cr0103221 Abstract
(http://dx.doi.org/10.1021/cr0300946) .
3. P. v. R. Schleyer, "Introduction: Delocalization-π and σ (Editorial)", Chemical Reviews, 2005, 105,
3433-3435. DOI: 10.1021/cr030095y Abstract (http://dx.doi.org/10.1021/cr030095y) .
4. CCXI.—Polynuclear heterocyclic aromatic types. Part II. Some anhydronium bases James Wilson
Armit and Robert Robinson Journal of the Chemical Society, Transactions, 1925, 127, 1604 - 1618
Abstract (http://dx.doi.org/10.1039/CT9252701604)
5. APPLICATION OF THE OCTET THEORY TO SINGLE-RING AROMATIC COMPOUNDS Ernest
C. Crocker J. Am. Chem. Soc.; 1922; 44(8) pp 1618 - 1630; Abstract
(http://dx.doi.org/10.1021/ja01429a002)
6. The structure of cycloid hydrocarbons Henry Edward Armstrong Proceedings of the Chemical
Society (London), 1890, 6, 95 - 106 Abstract (http://dx.doi.org/10.1039/PL8900600095)
7. A Double-Twist Möbius-Aromatic Conformation of [14]Annulene Henry S. Rzepa Org. Lett.; 2005;
7(21) pp 4637 Abstract (http://dx.doi.org/10.1021/ol0518333)
Topics in organic chemistry
Aromaticity Covalent bonding Functional groups Nomenclature Organic compounds Organic reactions
Organic synthesis Publications Spectroscopy Stereochemistry
List of organic compounds
Category: Aromatic compounds
This page was last modified 05:20, 14 November 2006.
All text is available under the terms of the GNU Free Documentation License. (See Copyrights for
details.)
Wikipedia® is a registered trademark of the Wikimedia Foundation, Inc.
Benzene - Wikipedia, the free encyclopedia
From Wikipedia, the free encyclopedia
Benzene, also known as benzol, is an organic chemical
compound with the formula C6H6. It is sometimes
abbreviated PhH. Benzene is a colorless and flammable
liquid with a sweet smell and a relatively high melting
point. It is carcinogenic and its use as additive in
gasoline is now limited, but it is an important industrial
solvent and precursor in the production of drugs,
plastics, synthetic rubber, and dyes. Benzene is anatural constituent of crude oil, but it is usually
synthesized from other compounds present in
petroleum. Benzene is an aromatic hydrocarbon and
Molecular formula
the second [n]-annulene ([6]-annulene).
2 Structure3 Substituted benzene derivatives
4.1 Catalytic reforming
4.2 Toluene hydrodealkylation
Density and phase
0.8786 g/cm³, liquid
4.3 Toluene disproportionation4.4 Steam cracking
Solubility in water
1.79 g/L (25 °C)
5.5 °C (278.6 K)
5.1 Early uses5.2 Current uses of benzene
80.1 °C (353.2 K)
6 Reactions of benzene
0.652 cP at 20 °C
7 Health effects8 References
Benzene has been the subject of many studies by many
famous scientists ranging from Michael Faraday to
Linus Pauling. In 1825 Faraday reported its isolation
EU classification
from oil gas and gave it the name bicarburet of
hydrogen. In 1833, Eilhard Mitscherlich produced it
Toxic (T)
via the distillation of benzoic acid (from gum benzoin)and lime. Mitscherlich gave the compound the name
benzin. In 1845, Charles Mansfield, working under
August Wilhelm von Hofmann, isolated benzene from
coal tar. Four years later, Mansfield began the first
Benzene - Wikipedia, the free encyclopedia
industrial-scale production of benzene, based on the
R45, R46, R11, R36/38,
coal-tar method.
R48/23/24/25, R65
Autoignition temperature 561 °C
Main article: aromaticity
The formula of benzene (C6H6) mystified scientists
who could not figure out its structure. Friedrich August Related
Kekulé von Stradonitz was the first to deduce the ring
structure of benzene. An often-repeated story claims
that after years of studying carbon bonding, benzene
Related compounds
and related molecules, he dreamt one night of the
Except where noted otherwise, data are given for
Ouroboros, a snake eating its own tail, and that upon
materials in their standard state (at 25°C, 100 kPa)
waking he was inspired to deduce the ring structure of
Infobox disclaimer and references
benzene. Other common tale is he obtained theinspiration from the figure of an hexagon in a tabern sign in Germany. However, the story first appeared inthe Berichte der Durstigen Chemischen Gesellschaft (Journal of the Thirsty Chemical Society), a parody ofthe Berichte der Deutschen Chemischen Gesellschaft, which appeared annually in the late-19th century onthe occasion of the congress of German chemists; accordingly, it is probably to be treated withcircumspection.
While his (more formal) claims were well-publicized and accepted, by the early-1920s Kekulé's biographercame to the conclusion that Kekulé's understanding of the tetravalent nature of carbon bonding depended onthe previous research of Archibald Scott Couper (1831-1892); furthermore, Josef Loschmidt (1821-1895)had earlier posited a cyclic structure for benzene as early as 1861. The cyclic nature of benzene was finallyconfirmed by the eminent crystallographer Kathleen Lonsdale.
Benzene presents a special problem in that, to account for all the bonds, there must be alternating doublecarbon bonds:
Using X-ray diffraction, researchers discovered that all of the carbon-carbon bonds in benzene are of thesame length of 140 picometres (pm). The C-C bond lengths are greater than a double bond (134pm) butshorter than a single bond (147pm). This intermediate distance is explained by electron delocalization: theelectrons for C-C bonding are distributed equally between each of the six carbon atoms. One representationis that the structure exists as a superposition of so-called resonance structures, rather than either formindividually. This delocalisation of electrons is known as aromaticity, and gives benzene great stability. Thisenhanced stability is the fundamental property of aromatic molecules that differentiates them from moleculesthat are non-aromatic. To reflect the delocalised nature of the bonding, benzene is often depicted with acircle inside a hexagonal arrangement of carbon atoms:
Benzene - Wikipedia, the free encyclopedia
As is common in organic chemistry, the carbon atoms in the diagram above have been left unlabeled.
Benzene occurs sufficiently often as a component of organic molecules that there is a Unicode symbol withthe code 232C to represent it:
?Many fonts do not have this Unicode character, so a browser may not be able to display it correctly.
Substituted benzene derivatives
Main article: Aromatic hydrocarbons
Many important chemicals are derived from benzene, wherein with one or more of the hydrogen atoms isreplaced with another functional group. Examples of simple benzene derivatives are phenol, toluene, andaniline, abbreviated PhOH,PhMe, and PhNH2, respectively. Linking benzene rings gives biphenyl,C6H5-C6H5. Further loss of hydrogen gives "fused" aromatic hydrocarbons, such naphthalene andanthracene. The limit of the fusion process is the hydrogen-free material graphite.
In heterocycles, carbon atoms in the benzene ring are replaced with other elements. The most importantderivatives are the rings containing nitrogen. Replacing one CH with N gives the compound pyridine,C5H5N. Although benzene and pyridine are structurally related, benzene cannot be converted into pyridine.
Replacement of a second CH bond with N gives, depending on the location of the second N, pyridazine,pyrimidine, and pyrazine.
Trace amounts of benzene may result whenever carbon-rich materials undergo incomplete combustion. It isproduced in volcanoes and forest fires, and is also a component of cigarette smoke.
Up until World War II, most benzene was produced as a byproduct of coke production in the steel industry.
However, in the 1950s, increased demand for benzene, especially from the growing plastics industry,necessitated the production of benzene from petroleum. Today, most benzene comes from the petrochemicalindustry, with only a small fraction being produced from coal.
Three chemical processes contribute equally to industrial benzene production: catalytic reforming, toluenehydrodealkylation, and steam cracking.
In catalytic reforming, a mixture of hydrocarbons with boiling points between 60-200 °C is blended with
Benzene - Wikipedia, the free encyclopedia
hydrogen gas and then exposed to a bifunctional platinum chloride or rhenium chloride catalyst at 500-525°C and pressures ranging from 8-50 atm. Under these conditions, aliphatic hydrocarbons form rings and losehydrogen to become aromatic hydrocarbons. The aromatic products of the reaction are then separated fromthe reaction mixture by extraction with any one of a number of solvents, including diethylene glycol orsulfolane, and benzene is then separated from the other aromatics by distillation.
Toluene hydrodealkylation converts toluene to benzene. In this process, toluene is mixed with hydrogen,then passed over a chromium, molybdenum, or platinum oxide catalyst at 500-600 °C and 40-60 atmpressure. Sometimes, higher temperatures are used instead of a catalyst. Under these conditions, tolueneundergoes dealkylation according to the chemical equation:
C6H5CH3 + H2 C6H6 + CH4
Typical reaction yields exceed 95%. Sometimes, xylene and heavier aromatics are used in place of toluene,with similar efficiency.
Where a chemical complex has similar demands for both benzene and xylene, then toluene
disproportionation (TDP) may be an attractive alternative. Broadly speaking 2 toluene molecules are reacted
and the methyl groups rearranged from one toluene molecule to the other, yielding one benzene molecule
and one xylene molecule.
Given that demand for para-xylene (p-xylene) substantially exceeds demand for other xylene isomers, a
refinement of the TDP process called Selective TDP (STDP) may be used. In this process, the xylene stream
exiting the TDP unit is approximately 90% paraxylene.
Steam cracking is the process for producing ethylene and other olefins from aliphatic hydrocarbons.
Depending on the feedstock used to produce the olefins, steam cracking can produce a benzene-rich liquidbyproduct called pyrolysis gasoline. Pyrolysis gasoline can be blended with other hydrocarbons as a gasolineadditive, or distilled to separate it into its components, including benzene.
Early uses
In the 19th and early-20th centuries, benzene was used as an after-shave lotion and for douches because ofits pleasant smell. Prior to the 1920s, benzene was frequently used as an industrial solvent, especially fordegreasing metal. As its toxicity became obvious, benzene has been supplanted by other solvents.
In 1903, Lugwig Roselius popularized the use of benzene to decaffeinate coffee. This discovery lead to theproduction of Sanka, -ka for kaffein. This process was later discontinued.
As a petrol additive, benzene increases the octane rating and reduces knocking. Consequently, petrol oftencontained several percent benzene before the 1950s, when tetraethyl lead replaced it as the most widely-usedantiknock additive. However, with the global phaseout of leaded petrol, benzene has made a comeback as agasoline additive in some nations. In the United States, concern over its negative health effects and the
Benzene - Wikipedia, the free encyclopedia
possibility of benzene entering the groundwater have led to stringent regulation of petrol's benzene content,with values around 1% typical. European petrol specifications now contain the same 1% limit on benzenecontent.
Current uses of benzene
Today benzene is mainly used as an intermediate to make other chemicals. Its most widely-producedderivatives include styrene, which is used to make polymers and plastics, phenol for resins and adhesives(via cumene), and cyclohexane, which is used in the manufacture of Nylon. Smaller amounts of benzene areused to make some types of rubbers, lubricants, dyes, detergents, drugs, explosives and pesticides.
In laboratory research, toluene is now often used as a substitute for benzene. The solvent-properties of thetwo are similar but toluene is less toxic and has a wider liquid range.
Benzene has been used as a basic research tool in a variety of experiments including analysis of atwo-dimensional gas.
Reactions of benzene
Electrophilic aromaticsubstitution is a generalmethod of derivatizingbenzene. Benzene issufficiently nucleophilicthat it undergoessubstitution by acyliumions or alkyl carbocationsto afford give substitutedderivatives.
The Friedel-Crafts acylation is a specificexample of electrophilic aromaticsubstitution. The reaction involves theacylation of benzene (or many otheraromatic rings) with an acyl chloride using astrong Lewis acid catalyst such as aluminiumchloride.
Like the Friedel-Craftsacylation, the Friedel-Craftsalkylation involves thealkylation of benzene (andmany other aromatic rings)usng an alkyl halide in thepresence of a strong Lewisacid catalyst.
sulfonation.
Nitration: Benzene undergoes nitration with nitronioum ions (NO +
2 ) as the electrophile. Thus,
warming benzene with a combination of concentrated sulphuric and nitric acid givesnitrobenzene.
Hydrogenation: Benzene and derivatives convert to cyclohexane and derivatives when treated withhydrogen at high hydrogen pressures.
Benzene is an excellent ligand in the organometallic chemistry of low-valent metals. Importantexamples include the sandwich and half-sandwich complexes respectively Cr(C6H6)2 and
Benzene - Wikipedia, the free encyclopedia
Benzene exposure has serious health effects. Breathing high levels of benzene can result in death, while lowlevels can cause drowsiness, dizziness, rapid heart rate, headaches, tremors, confusion, and unconsciousness.
Eating or drinking foods containing high levels of benzene can cause vomiting, irritation of the stomach,dizziness, sleepiness, convulsions, rapid heart rate, and death.
The major effect of benzene from chronic (long-term) exposure is to the blood. Benzene damages the bonemarrow and can cause a decrease in red blood cells, leading to anemia. It can also cause excessive bleedingand depress the immune system, increasing the chance of infection.
Some women who breathed high levels of benzene for many months had irregular menstrual periods and adecrease in the size of their ovaries. It is not known whether benzene exposure affects the developing fetusin pregnant women or fertility in men.
Animal studies have shown low birth weights, delayed bone formation, and bone marrow damage whenpregnant animals breathed benzene.
The US Department of Health and Human Services (DHHS) classifies benzene as a human carcinogen.
Long-term exposure to high levels of benzene in the air can cause leukemia, a potentially fatal cancer of theblood-forming organs. In particular, Acute Myeloid Leukemia (AML) may be caused by benzene.
Several tests can show if you have been exposed to benzene. There is a test for measuring benzene in thebreath; this test must be done shortly after exposure. Benzene can also be measured in the blood; however,because benzene disappears rapidly from the blood, measurements are accurate only for recent exposures.
In the body, benzene is metabolized. Certain metabolites can be measured in the urine. However, this testmust be done shortly after exposure and is not a reliable indicator of how much benzene you have beenexposed to, since the same metabolites may be present in urine from other sources.
The US Environmental Protection Agency has set the maximum permissible level of benzene in drinkingwater at 0.005 milligrams per liter (0.005 mg/L). The EPA requires that spills or accidental releases into theenvironment of 10 pounds (4.5 kg) or more of benzene be reported to the EPA.
The US Occupational Safety and Health Administration (OSHA) has set a permissible exposure limit of 1part of benzene per million parts of air (1 ppm) in the workplace during an 8-hour workday, 40-hourworkweek. The short term exposure limit for airborne benzene is 5 ppm for 15 minutes.
In March 2006, the official Food Standards Agency in Britain conducted a survey of 150 brands of softdrinks. It found that four contained benzene levels above World Health Organization limits. The affectedbatches were removed from sale.
In recent history there have been many examples of the harmful health effects of benzene and its derivatives.
Toxic Oil Syndrome caused localised immune-suppression in Madrid in 1981 from people ingestingbenzene-contaminated olive-oil. Chronic Fatigue Syndrome has also been highly correlated with people whoeat "denatured" food that use solvents to remove fat or contain benzoic acid.
Workers in various industries that make or use benzene may be at risk for being exposed to high levels ofthis carcinogenic chemical. Industries that involve the use of benzene include the rubber industry, oil
Benzene - Wikipedia, the free encyclopedia
refineries, chemical plants, shoe manufacturers, and gasoline related industries. In 1987, OSHA estimatedthat about 237,000 workers in the United States were potentially exposed to benzene, and it is not known ifthis number has substantially changed since then.
Water and soil contamination are important pathways of concern for transmission of benzene contact. In theU.S. alone there are approximately 100,000 different sites which have benzene soil or groundwatercontamination. In 2005, the water supply to the city of Harbin in China with a population of almost ninemillion people, was cut off because of a major benzene exposure. Benzene leaked into the Songhua River,which supplies drinking water to the city, after an explosion at a China National Petroleum Corporation(CNPC) factory in the city of Jilin on 13 November.
Main article: benzene in soft drinks
Archibald Scott Couper, On a New Chemical Theory, Philosophical Magazine 16, 104-116 (1858)Josef Loschmidt, Chemische Studien I, Carl Gerold's Sohn, Vienna (1861),Josef Loschmidt, Chemische Studien I, Aldrich Chemical Co, Milwaukee (1989), catalog no.
Z-18576-0, and (1913) catalog no. Z-18577-9Kathleen Lonsdale, "The Structure of the Benzene Ring in Hexamethylbenzene," Proceedings of theRoyal Society 123A: 494 (1929).
Kathleen Lonsdale, "An X-Ray Analysis of the Structure of Hexachlorobenzene, Using the FourierMethod," Proceedings o
ATSDR - Case Studies in Environmental Medicine: Benzene Toxicity(http://www.atsdr.cdc.gov/HEC/CSEM/benzene/)Benzene (http://www.phc.vcu.edu/Feature/oldfeature/benzene/index.html)Benzene Material Safety Data Sheet (http://www.hazard.com/msds/f2/bqv/bqvjq.html)International Chemical Safety Card 0015(http://www.ilo.org/public/english/protection/safework/cis/products/icsc/dtasht/_icsc00/icsc0015.htm)National Pollutant Inventory - Benzene(http://www.npi.gov.au/database/substance-info/profiles/12.html)NIOSH Pocket Guide to Chemical Hazards (http://www.cdc.gov/niosh/npg/npgd0049.html)IARC Monograph: "Benzene" (http://www-cie.iarc.fr/htdocs/monographs/suppl7/benzene.html)
CID 241 (http://pubchem.ncbi.nlm.nih.gov/summary/summary.cgi?cid=241) from PubChemComputational Chemistry Wiki (http://www.compchemwiki.org/index.php?title=Benzene)Couper and Carbon bonds(http://classes.yale.edu/chem125a/125/history99/5Valence/Couper/Couper.html)Dept. of Health and Human Services: TR-289: Toxicology and Carcinogenesis Studies of Benzene(http://ntp.niehs.nih.gov/index.cfm?objectid=0707525C-0F07-05BF-A16CAC7B0ECC97B5)Kekule, Couper and dreams of Benzene(http://classes.yale.edu/chem125a/125/history99/5Valence/Kekule/Kekule.html)Loschmidt's Benzene structure (http://www.physicstoday.org/pt/vol-54/iss-3/captions/p45cap4.html)video podcast (http://www.ch.ic.ac.uk/video/index.rss) (Sir john Cadogan giving a lecture on Benzenesince Faraday, in 1991)benzene 3D view and pdb-file(http://www.welsch.com/e/index.php?chap=6_1&modulekey=wpmoleculeviewer&specialmol=71-43-2Link page to external chemical sources.
Benzene - Wikipedia, the free encyclopedia
Categories: Annulenes Simple aromatic rings IARC Group 1 carcinogens Soil contamination Solvents Aromatic hydrocarbons Water pollution Immunotoxins
This page was last modified 05:36, 18 November 2006.
All text is available under the terms of the GNU Free Documentation License. (See Copyrights for
details.)
Wikipedia® is a registered trademark of the Wikimedia Foundation, Inc.
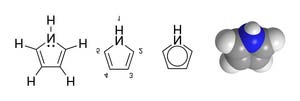
Pyrrole - Wikipedia, the free encyclopedia
From Wikipedia, the free encyclopedia
Pyrrole, or pyrrol, is a heterocyclic aromatic organic
compound, a five-membered ring with the formula
C4H5N.
Pyrroles are components of larger aromatic rings, including the porphyrins of heme, the chlorins and bacteriochlorins of chlorophyll, and the corrin ring of
vitamin B12.
Pyrrole has very low basicity compared to amines and other aromatic compounds like pyridine where the ring
nitrogen is not bonded to a hydrogen atom. This is
because the lone pair of electrons of the nitrogen atom
becomes delocalized in the aromatic ring.
Pyrrole undergoes electrophilic substitution
predominantly at the 2 and 5 positions, though the
substitution product at positions 3 and 4 is obtained in
Disclaimer and references
low yields. Pyrrole compounds can also participate in cycloaddition (Diels-Alder) reactions under certain conditions, such as Lewis acid catalysis, heating, or high pressure.
In a 1994 report released by five top cigarette companies, pyrrole is one of the 599 additives to cigarettes. Its use or purpose, however, is unknown, like most cigarette additives. [1](http://quitsmoking.about.com/cs/nicotineinhaler/a/cigingredients.htm)
PolypyrroleIndole, a derivative with a fused benzene ring.
Thiophene, an analog with a sulfur instead of the nitrogen atom.
Furan, an analog with an oxygen instead of the nitrogen.
Phosphole, a non-aromatic phosphorus analog.
Arsole, a moderately-aromatic arsenic analog.
Pyrrolidine, the saturated hydrogenated derivativeSimple aromatic ringsPyroluria
General Synthesis and Reactivity of Pyrrole(http://chemistry.tidalswan.com/index.php?title=Heteroaromatics#Pyrrole_.28Azole.29) Synthesis of pyrroles (overview of recent methods)(http://www.organic-chemistry.org/synthesis/heterocycles/pyrroles.shtm) Substitution reaction mechanisms of nitrogen-containing heteroaromatics(http://users.ox.ac.uk/ mwalter/web_05/year2/arom2/hetarom_rxn_mech.shtml)
Pyrrole - Wikipedia, the free encyclopedia
Link page to external chemical sources.
1. Loudon, Marc G. (2002). "Chemistry of Naphalene and the Aromatic Heterocycles.", Organic
Chemistry, Fourth Edition, New York: Oxford University Press, 1135-1136. ISBN 0-19-511999-1.
Categories: Nitrogen heterocycles Simple aromatic rings Amines Amine stubs
This page was last modified 18:27, 27 September 2006.
All text is available under the terms of the GNU Free Documentation License. (See Copyrights for
details.)
Wikipedia® is a registered trademark of the Wikimedia Foundation, Inc.
Furan - Wikipedia, the free encyclopedia
From Wikipedia, the free encyclopedia
Furan, also known as furane and furfuran, is a heterocyclic
organic compound, produced when wood, especially
oxole, furfuran, divinyl oxide
pine-wood, is distilled. Furan is a clear, colorless, very volatile
Chemical formula C
and highly flammable liquid with a boiling point close to room
temperature. It is toxic and may be carcinogenic. Catalytic
hydrogenation (see redox) of furan with a palladium catalyst
Furan is aromatic because one of the lone pairs of electrons on the oxygen atom is delocalized into the ring, creating a 4n+2
aromatic system (see Hückel's rule) similar to benzene. This
aromatic system forms two rings of electrons above and below the flat structure with no discrete double bonds. The other lone pair of electrons of the oxygen atom extends in the plane of the flat ring system.
1 Synthesis2 Reactions
Except where noted otherwise, data are given for
materials in their standard state (at 25 °C, 100 kPa)
Infobox disclaimer and references
A classic furan organic synthesis is the Feist-Benary synthesis.
One of the most simple synthesis methods for furans is the reaction of 1,4-diketones with diphosphorus pentoxide (P2O5) in the Paal-Knorr Synthesis. It is interesting that the thiophene
formation reaction of 1,4-diketones with lawesson's reagent also forms furans as side products.
Due to its aromaticity, furan's behavior is quite dissimilar to that of the more typical heterocyclic ethers such as tetrahydrofuran. It is considerably more reactive than benzene in electrophilic substitution reactions.
Furan is a diene in Diels-Alder reactions with electron-deficient dienophiles such as ethyl (E)-3-nitroacrylate.[1] The reaction product is a mixture of isomers with preference for the endo isomer:
Furan - Wikipedia, the free encyclopedia
Furans are hydrogenated to dihydrofurans and tetrahydrofurans.
Tetrahydrofuran (THF), the fully hydrogenated analog of furan and a common solvent.
Pyrrole, the nitrogen analog of furan.
Thiophene, the sulfur analog of furan.
Selenophene, the selenium analog of furan.
Tellurophene, the tellurium analog of furan.
Benzofuran, furan with a fused benzene ring.
Dibenzofuran, a compound class similar to dibenzodioxins.
Simple aromatic rings
Computational Chemistry Wiki (http://www.compchemwiki.org/index.php?title=Furan) Recent synthetic methods(http://www.organic-chemistry.org/synthesis/heterocycles/furans/furans.shtm) Link page to external chemical sources.
1. The oxanorbornene approach to 3-hydroxy, 3,4-dihydroxy and 3,4,5-trihydroxy derivatives of
2-aminocyclohexanecarboxylic acid Masesane I, Batsanov A, Howard J, Modal R, Steel P Beilstein
Journal of Organic Chemistry, 2006 2:9 Online Article
(http://bjoc.beilstein-journals.org/content/pdf/1860-5397-2-9.pdf)
Categories: Furans Simple aromatic rings
This page was last modified 18:34, 19 November 2006.
All text is available under the terms of the GNU Free Documentation License. (See Copyrights for
details.)
Wikipedia® is a registered trademark of the Wikimedia Foundation, Inc.
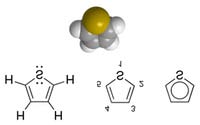
Thiophene - Wikipedia, the free encyclopedia
From Wikipedia, the free encyclopedia
Thiophene is an aromatic
heterocyclic compound
consisting of four carbon
atoms and one sulfur atom
in a five-membered ring.
Compounds analogous to
thiophene include furan
and pyrrole where the S
atom is replaced by O and
NH, respectively. Thiophene was discovered
by Viktor Meyer in 1871 as a contaminant in
Molecular formula C4H4S
benzene. Related to
benzothiophene and
dibenzothiophene,
containing the thiophene ring fused with one and
two benzene rings,
Density and phase 1.051 g/ml, liquid
Immiscible with water
soluble in most organic solvents
External MSDS (http://physchem.ox.ac.uk/MSDS/TH/thiophene.html)
occurrence4 See also
EU classification
At room temperature, thiophene is a colorless
liquid with a mildly
Supplementary data page
pleasant odor reminiscent
of benzene, with which
thiophene shares some
Solid, liquid, gas
Thiophene is considered
Thiophene - Wikipedia, the free encyclopedia
aromatic, although
theoretical calculations
Related thioethers
suggest that the degree of
aromaticity is less than that
Related compounds
participation of the lone
Except where noted otherwise, data are given for
electron pairs on sulfur in
materials in their standard state (at 25 °C, 100 kPa)
Infobox disclaimer and references
the delocalized pi electronsystem is significant. As a consequence of its aromaticity, thiophene does not exhibit the properties seen for conventional thioethers. For example the sulfur atom is not alkylated by methyl iodide. Although the sulfur atom is unreactive, the flanking CH centers are susceptible to attack by electrophiles. The high reactivity of thiophene toward sulfonation is the basis for the separation of thiophene from benzene. Due to the mere 4 °C difference intheir boiling points at ambient pressure, thiophene and benzene are difficult to separate by distillation. Treatment of thiophene-benzene mixtures with sulfuric acid results in preferential sulfonation of the thiophene to give water-soluble thiophene sulfonic acid.
Thiophenes are important heterocyclic compounds and are recurring building blocks in organic chemistry with applications in pharmaceuticals. For example, thiophenes are used as synthetic intermediates, taking advantage of the susceptability of the carbon atoms adjacent to S toward electrophilic reactions. Desulfurization of the resulting ring using Raney nickel affords 1,4-disubstituted butanes. The polymer formed by linking thiophene through its 1,5 positions is called polythiophene. Polythiophenes become electrically conductive upon partial oxidation, i.e. they become "organic metals".
Thiophene is used as a denaturant for ethanol with which it forms an azeotrope.
Synthesis and occurrence
Reflecting their high stabilities, thiophenes arise from many reactions involving sulfur sources and hydrocarbons, especially unsaturated ones, e.g. acetylenes and elemental sulfur. Thiophenes are classically prepared by the reaction of 1,4-diketones with sulfiding reagents such as Lawesson's reagent or P4S10. Specialized thiophenes can be synthesized via the Gewald reaction, which involves the condensation of two esters in the presence of elemental suflur.
Thiophene and its derivatives occur in petroleum, sometimes in concentrations up to 1-3 %. The thiophenic content of liquids from oil and coal is removed via the hydrodesulfurization (HDS) process. In HDS, the liquid or gaseous feed is passed over a special form of molybdenum disulfide under a pressure of H2. Thiophenes undergo hydrogenolysis to form hydrocarbons and hydrogen sulfide. Thus, thiophene itself is converted to butane and H2S. More prevalent and more problematic in petroleum are benzothiophene and dibenzothiophene.
Pyrrole, an analog with a NH instead of the sulfur atom.
Furan, an analog with an oxygen instead of the sulfur atom.
Simple aromatic rings
Thiophene - Wikipedia, the free encyclopedia
International Chemical Safety Card 1190
(http://www.ilo.org/public/english/protection/safework/cis/products/icsc/dtasht/_icsc11/icsc1190.htm)
[1] (http://pubs.acs.org/journals/chreay/index.html) Roncali, J., "Conjugated Poly(thiophenes):
Synthesis, Functionalization, and Applications", Chemical Reviews, 1992, v. 92, p 711-738.
Link page to external chemical sources.
Categories: Thioethers Sulfur heterocycles Simple aromatic rings
This page was last modified 22:56, 13 August 2006.
All text is available under the terms of the GNU Free Documentation License. (See Copyrights for
details.)
Wikipedia® is a registered trademark of the Wikimedia Foundation, Inc.
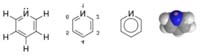
Pyridine - Wikipedia, the free encyclopedia
From Wikipedia, the free encyclopedia
Pyridine is a chemical compound with the formula C5H5N.
It is a liquid with a distinctively sour odour. Pyridine is a
simple and fundamentally important heterocyclic aromatic
organic compound that is structurally related to benzene,
wherein one CH group in the six-membered ring replaced
by a nitrogen atom. The pyridine ring occurs in many
important compounds, includine the nicotinamides.
Molecular formula
2 Pyridine as a solvent
colourless liquid
3 Role in chemical synthesis4 Preparation and occurrence
5 Safety and Environmental
6 Related compounds7 See also
Density and phase
0.9819 g/cm³, liquid
Solubility in water
Solubility in ethanol
0.94 cP at 20 °C
Pyridine has an equatorial lone pair of
electrons at the nitrogen atom. Because this
Standard enthalpy
lone pair is not delocalized into the aromatic
pi-system, pyridine is basic with chemical
of formation ΔfH°liquid
properties similar to tertiary amines. The pKa Standard enthalpy
of the conjugate acid is 5.30. Pyridine is
of combustion ΔcH°liquid
protonated by reaction with acids and forms
Standard molar entropy
a positively charged aromatic polyatomic ion S°
called pyridinium cation. The bond lengths
and bond angles in pyridine and the pyridinium ion are
almost identical.[1] because protonation does not affect the
EU classification
aromatic pi system.
Harmful (Xn)
Pyridine as a solvent
Pyridine is widely used as a versatile solvent being polar
but aprotic. It is fully miscible with a very broad range of solvents including hexane and water. The deuterated
R11, R20, R21, R22
pyridine, so called pyridine-d5 is a common solvent for 1H S-phrases
Pyridine - Wikipedia, the free encyclopedia
NMR spectroscopy.
Supplementary data page
Role in chemical synthesis
Structure andproperties
n, εr, etc.
Pyridine is important in industrial organic chemistry as both Thermodynamic
a fundamental building block and as a solvent and reagent
Solid, liquid, gas
in organic synthesis.[2] It is used as a solvent in
RTECS number, etc.
Pyridine-borane, C5H5NBH3 (m.p. 10–11 °C) is a mildreducing agent with improved stability vs NaBH
solvents and improved solubility in aprotic organic
solvents. Pyridine-sulfur trioxide, C
5H5NSO3 (mp 175 °C)
is a sulfonation agent used to convert alcohols to sulfonates,
Related compounds
which in turn undergo C-O bond scission upon reduction
with hydride agents.
Except where noted otherwise, data are given for
materials in their standard state (at 25 °C, 100 kPa)
It is also a starting material in the synthesis of compounds
Infobox disclaimer and references
used as an intermediate in making insecticides, herbicides, pharmaceuticals, food flavorings, dyes, rubber chemicals, adhesives, paints, explosives and disinfectants. Pyridine is also used as a denaturant for antifreeze mixtures, for ethyl alcohol, and for fungicides, and as a dyeing aid for textiles.
Pyridine is a good nucleophile with a donor number of 33.1. It is easily attacked by alkylating agents to give N-alkylpyridinium salts.
Preparation and occurrence
See also: Hantzsch pyridine synthesis
Pyridine is obtained from crude coal tar or is synthesized from acetaldehyde, formaldehyde and ammonia. Other examples of the pyridine class can be formed by the reaction of 1,5-diketones with ammonium acetatein acetic acid followed by oxidation. Pyridium salts can be obtained in the Zincke reaction.
Safety and Environmental
Pyridine is toxic with LD50 in rats (oral) of 891 mg kg–1. It is volatile and can be absorbed through skin, it is known to reduce male fertility and is considered carcinogenic as well. Common symptoms of exposure to pyridine include: headache, coughing, asthmatic breathing, laryngitis, nausea and vomiting. Though resistant to oxidation, pyridine is readily degraded by bacteria, releasing ammonium and carbon dioxide as terminal degradation products. [3]
Structurally or chemically related compounds are
DMAP is short for 4-dimethylaminopyridineBipyridine and viologen are simple polypyridine compounds consisting of two pyridine molecules joined by a single bond
Pyridine - Wikipedia, the free encyclopedia
Terpyridine, a molecule of three pyridine rings connected together by two single bonds.
Quinoline and Isoquinoline have pyridine and a benzene ring fused together.
Aniline is a benzene derivative with an attached NH2 group and NOT a pyridine
Diazines are compounds with one more carbon replaced by nitrogen such as Pyrazine and Pyramidine
Triazines are compounds with two more carbons replaced by nitrogen
2,6-Lutidine is a trivial name for 2,6-dimethylpyridine.
Collidine is the trivial name for 2,4,6-trimethylpyridine.
Pyridinium p-toluenesulfonate (PPTS) is a salt formed by proton exchange between pyridine and
p-toluenesulfonic acid
2-Chloropyridine is a toxic environmentally significant component of the breakdown of the pesticide
imidacloprid.
Simple aromatic rings6-Membered aromatic rings with one carbon replaced by another group: borabenzene, silabenzene, germanabenzene, stannabenzene, phosphorine, pyrylium salt
1. T. M. Krygowski, H. Szatyowicz, and J. E. Zachara J. Org. Chem. 2005 70(22) 8859 - 8865;
2. Sherman, A. R. "Pyridine" in Encyclopedia of Reagents for Organic Synthesis (Ed: L. Paquette)
2004, J. Wiley & Sons, New York. DOI:10.1002/047084289 (http://dx.doi.org/10.1002/047084289) .
3. Sims, G.K. and O'Loughlin, E.J. Degradation of pyridines in the environment. CRC Critical Reviews
in Environmental Control. (1989) 19(4):309-340.
International Chemical Safety Card 0323(http://www.ilo.org/public/english/protection/safework/cis/products/icsc/dtasht/_icsc03/icsc0323.htm) NIOSH Pocket Guide to Chemical Hazards (http://www.cdc.gov/niosh/npg/npgd0541.html) Computational Chemistry Wiki (http://www.compchemwiki.org/index.php?title=Pyridine) Examples of Pyridines(http://www.chemsuppliers.org/chemicals.php?mode=searchname&searchtext=Pyridine&page=0) Synthesis of pyridines (overview of recent methods)(http://www.organic-chemistry.org/synthesis/heterocycles/pyridines.shtm) Link page to external chemical sources.
Categories: Articles with unsourced statements Pyridines Simple aromatic rings Amines Solvents Foul-smelling chemicals
This page was last modified 18:48, 19 November 2006.
All text is available under the terms of the GNU Free Documentation License. (See Copyrights for
details.)
Wikipedia® is a registered trademark of the Wikimedia Foundation, Inc.
Source: http://www.thierrykarsenti.ca/sitesweb/peer_evaluation/vf/readings/phase1/mhehe_readings.pdf
Philadelphia University Department of Athletics Compliance Program and Table of Contents Letter from the Director of Athletics Mission Statement Department of Athletics Directory Important Contacts on Campus Division of Student Life- Organizational Chart Section I – General Policies and Procedures Work
Design of Group IIA Secreted/Synovial Phospholipase A2Inhibitors: An Oxadiazolone Derivative SuppressesChondrocyte Prostaglandin E2 Secretion Jean-Edouard Ombetta1., Natacha Thelier2., Chang Zhi Dong3, Ste´phanie Plocki3, Lydia Tsagris2, Franc¸ois Rannou2,4, France Massicot5, Atime´ Djimde´3, Elissar El-Hayek2, Yiming Shi3, Franc¸oise Heymans3, Nohad Gresh6, Caroline Chauvet2*