Wifiinschools.org.uk
Appl Biochem Biotechnol (2013) 169:1727–1751DOI 10.1007/s12010-012-0079-9
2.45 GHz Microwave Irradiation-Induced OxidativeStress Affects Implantation or Pregnancy in Mice,
Saba Shahin & Vineet Prakash Singh & Ritesh K. Shukla &Alok Dhawan & Ravi Kumar Gangwar &Surya Pal Singh & Chandra Mohini Chaturvedi
Received: 17 August 2012 / Accepted: 27 December 2012 /Published online: 22 January 2013
# Springer Science+Business Media New York 2013
Abstract The present experiment was designed to study the 2.45 GHz low-level microwave(MW) irradiation-induced stress response and its effect on implantation or pregnancy infemale mice. Twelve-week-old mice were exposed to MW radiation (continuous wave for2 h/day for 45 days, frequency 2.45 GHz, power density=0.033549 mW/cm2, and specificabsorption rate=0.023023 W/kg). At the end of a total of 45 days of exposure, mice weresacrificed, implantation sites were monitored, blood was processed to study stress parame-ters (hemoglobin, RBC and WBC count, and neutrophil/lymphocyte (N/L) ratio), the brainwas processed for comet assay, and plasma was used for nitric oxide (NO), progesterone andestradiol estimation. Reactive oxygen species (ROS) and the activities of ROS-scavenging
S. Shahin V. P. Singh C. M. Chaturvedi (*)Department of Zoology, Banaras Hindu University, Varanasi 221005, Indiae-mail:
[email protected]
S. Shahine-mail:
[email protected]
V. P. Singhe-mail:
[email protected]
R. K. Shukla A. DhawanIndian Institute of Toxicology Research, Lucknow 226 001, India
R. K. Shuklae-mail:
[email protected]
A. Dhawane-mail:
[email protected]
R. K. Gangwar S. P. SinghDepartment of Electronics Engineering, Indian Institute of Technology, Banaras Hindu University,Varanasi 221005, India
R. K. Gangware-mail:
[email protected]
S. P. Singhe-mail:
[email protected]
Appl Biochem Biotechnol (2013) 169:1727–1751
enzymes— superoxide dismutase, catalase, and glutathione peroxidase—were determined inthe liver, kidney and ovary. We observed that implantation sites were affected significantly inMW-irradiated mice as compared to control. Further, in addition to a significant increase inROS, hemoglobin (p<0.001), RBC and WBC counts (p<0.001), N/L ratio (p<0.01),DNA damage (p <0.001) in brain cells, and plasma estradiol concentration (p<0.05), asignificant decrease was observed in NO level (p<0.05) and antioxidant enzyme activ-ities of MW-exposed mice. Our findings led us to conclude that a low level of MWirradiation-induced oxidative stress not only suppresses implantation, but it may alsolead to deformity of the embryo in case pregnancy continues. We also suggest that MWradiation-induced oxidative stress by increasing ROS production in the body may lead toDNA strand breakage in the brain cells and implantation failure/resorption or abnormalpregnancy in mice.
Keywords Microwave radiation . Reactive oxygen species (ROS) . Nitric oxide . Antioxidantenzyme activity . Implantation failure
Microwaves (MW) are non-ionizing electromagnetic radiation (EMR) (wavelength rangingfrom 1 mm to 1 m and frequency between 0.3 and 300 GHz), which unlike ionizingradiation, do not contain sufficient energy to break the bond or chemically change thesubstances by ionization. In general, non-ionizing radiations are associated with two majorpotential hazards, i.e., electrical and biological. In recent times, the level of EMR in ourenvironment has increased manifold due to a large-scale expansion of communicationnetworks such as mobile phones, base stations, WLAN, Wi-Fi, Wi-MAX, etc. Radiationsemitted from these modern devices are reported to induce various types of biological effectswhich are of great concern to human health due to its increased use in daily life. MWradiation primarily increases the temperature of the biological system, i.e., thermal effects[but its nonthermal effects have also been noted and studied in detail Nonthermaleffects occur when the intensity of the MW radiation is sufficiently low so that the amount ofenergy involved would not significantly increase the temperature of a cell, tissue, or anorganism, but may induce some physical or biochemical changes [Prolonged exposure tolow intensity 2.45 GHz microwave radiation may affect the cholinergic activity in the rat [brain development in mice [], DNA breakage in rat brain ], and histone kinase activityin rat ], which results in neurological problems and reproductive disorders inaddition to changes in hematopoiesis of pregnant mice [and micronucleated erythrocytesin rats [The International Agency for Research on Cancer has also kept radiofrequencyelectromagnetic fields in the list of factors causing cancer to humans. Some studiesperformed in this context suggest that people heavily exposed to these radiations are moreprone to nonmalignant tumors It has been reported that mobile phone or cell phoneradiation (a type of MW radiation) causes changes in cognitive function [A Germanstudy has indicated an increase in cancer around base stations. Mobile phones use electro-magnetic radiation in a microwave range (2G—900/1,800 MHz, 3G—2,100 MHz frequencyband) which some believe may be harmful to human health. People living close to 2G andmostly 3G mobile phone masts or base stations frequently report symptoms of electromag-netic hypersensitivity such as dizziness, headaches, skin conditions, allergies, and manyother problems. Hardell and groups [] have reported the health implications of mobilephone exposure (800–2,200 MHz). They found that cell phone users had an increased risk of
Appl Biochem Biotechnol (2013) 169:1727–1751
malignant gliomas and higher rate of acoustic neuromas. The low-level nonthermal MWradiations or mobile phone radiations may propagate through the body and affect thereproductive system.
Nonthermal 2.45 GHz MW radiation may also act as one of the environmental stressors
responsible for increasing the production of reactive oxygen species (ROS) such as super-oxide (O •−
), hydrogen peroxide (H2O2), hydroxyl radical (•OH) and nitric oxide (NO•) in
the body which in turn are thought to be responsible for various pathological conditionsincluding metabolic disturbances affecting the growth and normal development of anorganism. Its production can also be increased in the oocyte microenvironment and uterinehorns in response to infection, inflammation, radiation, pollutants, etc. Therefore, increasedproduction of ROS is cytotoxic, while low levels are necessary for the regulation of severalkey physiological mechanisms. ROS-scavenging enzymes—superoxide dismutase (SOD),catalase (CAT) and glutathione peroxidase (GPx)—combinatorially play a major role inmaintaining the intracellular concentration of ROS. SOD, CAT and GPx together withglutathione (GSH) form the first line of defense against ROS. SOD catalyzes superoxideanion (O •−
) to O2 and H2O2, which is then reduced to H2O by the H2O2-scavenging
enzymes CAT and GPx [If H2O2 is not removed, it will cause oxidative damage tobiomolecules or can be converted to more damaging hydroxyl radical (•OH) species.
Therefore, the elimination of H2O2 is critical to the efficacy of SOD in reducing oxidativestress generated by ROS, whereas disruption of the antioxidant SOD leads to congenitalanomalies that are associated with increased oxidative DNA damage []. This will alsoresult in proliferation-impaired embryonic development and increased morbidity in theoffspring ].
ROS is a well-known factor that disrupts the hormonal communication between the brain,
pituitary, and the ovary. Thus, ROS interferes with both the maturation and the ovulationprocess of an egg along with the movement and implantation of a fertilized egg. High levelsof ROS and low antioxidant activity are responsible for decreased steroidogenesis and PCOScondition Cyclic production of ROS not only decreases ovarian function [butalso affects the dominant follicle selection [] and embryonic germ cell proliferation [Studies show that EMR penetrating the living organism may affect the free radical process-es/reaction within the cell [by altering the cell membrane potential. Nitric oxide (NO),which is a free radical gas and a ubiquitous signaling molecule produced by NO synthases,has been proposed to mediate many physiological functions including smooth musclerelaxation, vasodilation, neuronal signaling, immune response, regulation of embryonicdevelopment and implantation It is a potent relaxant of myometrial smooth muscle,and its upregulation during pregnancy is confirmed by the urinary excretion of cyclicguanosine monophosphate (cGMP) and nitrite–nitrate ]. NO can promote or inhibitimplantation depending on its critical level in the uterus and may also contribute as anantiplatelet agent during implantation It also stimulates the release and synthesis ofGnRH and may play a role in the induction of the steroid-induced LH surge. It is a potentialmediator of estrogen action on the hypothalamus and provokes estrogen-stimulated rapiduterine secretory response at the implantation site via cGMP. Under appropriate conditions,NO reacts with O •−
yielding peroxynitrite (ONOO−), a potent oxidant ], which further
decomposes to form the reactive hydroxyl radical. Moreover, peroxynitrite and its metabo-lite are capable of producing cytotoxicity by inducing lipid peroxidation, nitrosation ofseveral tyrosine molecules that regulate enzyme function, signal transduction and Na+channel inactivation. Nitrosation of tyrosine residues results in the formation of stableadduct nitrotyrosine [The decay of nitrotyrosine causes an increase in the nitrite(NO −
2 )/nitrate (NO3 ) ratio. The action of NO in a cell depends on its concentration, the
Appl Biochem Biotechnol (2013) 169:1727–1751
cellular redox state, the abundance of metals, protein thiols and low molecular weight thiols(glutathione) as well as other nucleophile targets [, ].
Electromagnetic radiation has been linked to a variety of adverse human health outcomes
including leukemia, brain tumors, genotoxic effects (DNA damage and micronucleation),neurological effects and neurodegenerative diseases, immune system deregulation, allergicand inflammatory responses, breast cancer in men and women, miscarriage and somecardiovascular effects. A number of studies have focused on microwave-induced biologicalresponses including oxidative stress , due to overproduction of ROS, but so far, thereis no comprehensive study describing the effects of 2.45 GHz low-level MW radiation onreproduction with special reference to implantation. However, it has been reported that MW-irradiated female mouse shows differential sensitivity during the successive stages of theestrous cycle with greater radiosensitivity during estrus than during diestrus []. This studyalso indicated that the pregnant mouse exposed to MW radiation with an average absorbeddose ranging from 3 to 8 cal/g body weight at a temperature–humidity index of 71.6 showsevidence of teratogenesis, particularly in the form of a brain hernia or exencephaly inaddition to gross anomalies including hemorrhage, resorptions, stunting and even fetaldeath. Further, studies related to EMR radiations revealed that exposure to 2.45 GHzcontinuous wave (CW) microwave affects embryo-fetal development [uteroplacentalblood flow, progesterone and PGF2 levels ]. But some investigators have reported that2.45 GHzMW radiation exposure at different power densities had no remarkable effect onpregnancy or the development of the preimplantation embryo [].
In view of the available reports, it is evident that high ROS may affect ovarian develop-
ment/function adversely, and MW exposure at higher specific absorption rate (SAR) valuemay induce adverse embryonic growth if any. However, in our preliminary published study[we found that 2.45 GHz low-level CW microwave radiation affects embryo implan-tation sites and caused DNA damage in the brain cell of mice, but yet no information isavailable regarding the mechanism of action of low-level MW radiation on implantation/pregnancy especially at lower SAR value. Hence, on the basis of our previous study andavailable reports, the present experiment was designed to examine whether or not the2.45 GHz low-level MW irradiation results in oxidative stress by altering ROS and ROS-scavenging enzymes could affect the implantation or pregnancy in mice.
Materials and Methods
Animals and Microwave Irradiation
Twelve-week-old female mice (Parkes strain) were obtained from the mice colony of ourlaboratory maintained under a light/dark cycle (LD12:12). Animals were supplied with food(standard rodent food pellets supplied by Pashu Aahar Kendra, Varanasi, India) and tapwater ad libitum. Twelve randomly selected mice were divided into two groups(control and experimental) of six mice each (N = 6). Experimental animals wereexposed to 2.45 GHz CW low-level microwave radiation (2 h/day, from 09:00 to 11:00 hoursfor 45 days) as described below.
Twenty days after MW exposure (pre-mating phase), mice were numbered and mating
was set by keeping two females with one male for 5 days. During this mating phase, femaleswere separated from the male only during 2 h of the MW exposure period and then kept backin the regular cage with the same male to avoid Bruce effect. After 5 days, pregnant femaleswere not kept with the males but MW exposure was continued as above for another 20 days
Appl Biochem Biotechnol (2013) 169:1727–1751
(post-mating phase). The control animals were subjected to sham exposure with similar pre-mating/mating/post-mating condition. The rectal temperature of both groups was measuredbefore and after the exposure during the first and last 3 days of the pre-mating phase and thenfinally during the last 3 days of the experiment or post-mating phase. The experiment wasconducted in accordance with institutional practice and within the framework of the revisedAnimals (Scientific procedures) Act of 2002 of the Government of India on Animal Welfare.
The whole experiment was repeated again to confirm the results.
The Analog Signal Generator microwave source (Model no. E 8257D PSG, manufacturedby Agilent Technologies, USA) covering a frequency range from 250 kHz to 20 GHz, acoaxial attenuator, microwave amplifier (model no. 8349B Hewlett Packard Co., USA),coaxial to waveguide transition, 20 dB cross coupler and E-plane bend were used to delivermicrowave power to pyramidal horn antenna. The maximum output power delivered to thehorn antenna from the amplifier as measured by the power sensor (Model no. 836A,manufactured by Agilent Technologies) and power meter (Model no. 8481H, HewlettPackard Co., USA) having a range from 30 μW to 3 W was 19.8 dBm. A silver-polishedbrass conductor was used to fabricate the pyramidal horn antenna. The throat and mouthdimensions of the antenna are 7.2 cm×3.2 cm and 9.0 cm×5.0 cm, respectively. Theaxial length of the antenna from the throat is 10.0 cm. The gain of the horn antenna (Gt)was measured by the comparison method and is found to be 4.0657 dB. The distancebetween the pyramidal horn and the midplane of the body of the animal (assuming thebody length to remain horizontal during exposure) was estimated through far fieldcriterion (R≥2D2/λ) to be 25 cm. The cross-sectional dimensions of the animal cage inE- and H-planes (electric and magnetic field planes, respectively) were designed on thebasis of 1.24 and 1.69 dB beam widths in corresponding planes of the horn, respectively.
For this purpose, the horn radiation patterns were also measured at 2.45 GHz in E- andH-planes ].
The dimensions of the animal cage are 19.2 cm×17.6 cm×15 cm. The cage was designed
in such a way that it contains six compartments of equal size (Fig. The dimensions ofeach compartment are 6 cm×8 cm×15 cm accommodating a single mouse each at a time.
The cage and its partitions were made up of pine wood having very low dielectric constant.
Small circular holes of 1 cm diameter were drilled on the side and the partition walls of thecage for air ventilation. The upper portion of the cage remained open to keep the animalsaerated during exposure. The upper portion of the side and partition walls and the base of thecage were covered by carbon-impregnated styrofoam microwave absorber to reduce elec-tromagnetic scattering. Each animal was kept in a separate compartment of the cagethroughout the exposure period. The partitions were made in such a way that mice remainrestrained in the cage during the period of microwave irradiation and were exposed parallelto the E field. The animal cage was placed on the microwave absorber and also covered fromall four sides by microwave absorbers to minimize scattering/reflections. The cage was alsotied to the absorber unit to avoid any change in the position of the cage with reference to thehorn antenna. The temperature in the chamber was maintained at 25–27 °C throughout theexperiment by circulating air. Mice were exposed for 2 h, i.e., from 09:00 to 11:00 daily for45 days continuously. The 20-dB cross coupler and power meter with power sensor wereused to measure the power input to the antenna and reflections from it. Power transmittedfrom the antenna was estimated by subtracting the power reflected from the antenna when itfaces towards free space from the measured input power. This way, power transmitted by the
Appl Biochem Biotechnol (2013) 169:1727–1751
Power Sensor with
Power meter/ Matched Load
Waveguide Transition
Pyramidal
horn
Power Sensor with Power meter/ Matched Load
Holes for
ventilation
Fig. 1 Diagrammatic representation of the microwave exposure setup and the position of animal cages duringmicrowave exposure. Mice were exposed to 2.45 GHz low-level MW radiation for 2 h/day continuously for45 days by using microwave source and pyramidal horn antenna
antenna was estimated which is equal to 64.776 mW. The power density was computedusing the following formula:
Power Density ¼ PtGt
where Pt is the power transmitted into the cage, Gt is the gain of the horn, and R is thedistance between the horn aperture and midplane of the body of the mouse in the exposurecage. The experimental group of mice was exposed to 2.45 GHz microwave radiation at apower density of 0.033549 mW/cm2 and SAR of 0.023023 W/kg, which is comparativelymuch lower in context with the SAR of other earlier studies. The SAR was estimated forbody length parallel to the electric field, as per actual placement of mice The 0.033549-mW/cm2 exposure does not cause any elevation in rectal temperature. The average rectaltemperatures of the mice observed were 35.81±0.2 °C (control group) and 35.94±0.2 °C(experimental group) before and 35.91 ± 0.2 °C (control group) and 36.05 ± 0.2 °C(experimental group) just after the termination of exposure.
Collection of Blood and Tissue Samples
After the termination of 45 days exposure, mice were sacrificed by decapitation and bloodwas collected in heparinized tubes to study hematological parameters. Brain was dissectedout immediately after decapitation and processed for comet assay. Liver, kidney, and ovary
Appl Biochem Biotechnol (2013) 169:1727–1751
were removed and, after washing with ice-cold sterile physiological saline solution, pro-cessed for ROS measurement and antioxidant assay.
Implantation Sites
Sacrificed animals were dissected for visualization of the implantation sites and distributionof embryos in the uterine horns.
Blood was used to determine the total number of erythrocytes, leucocytes, and neutrophil/lymphocyte ratio (N/L ratio) by the hemocytometer method. The hemoglobin content in theblood was measured by a hemometer (Sahli's method).
For comet assay, brain tissue was processed according to the method of Hartmann et al. ]as described by Patel et al. ] for a single-cell suspension preparation. Briefly, brain wasplaced in 1 ml chilled mincing solution (Hank's balanced salt solution, with 20 mM EDTAand 10 % DMSO) in a Petri dish and chopped into small pieces with a pair of scissors. Thepieces were allowed to settle and the supernatant containing the single cell suspension wastaken. Normal melting agarose (NMA, 1.0 %) was prepared in Milli-Q water, microwaved,and kept at 60 °C. Conventional glass slides were then dipped into molten NMA up to twothirds of their frosted end, the lower surface was wiped clean and the slides were left to dry.
On this first layer, 80 μl of diluted sample (100 μl cell suspension mixed with 100 μl of 1 %low melting point agarose, LMA) was added to form the second layer. A cover slip (size24 mm×60 mm) was placed gently to evenly spread the cells in the agarose. The slides werekept on ice for 5 min to allow the gel to solidify. The cover slips were removed and a thirdlayer of 0.5 % LMA (90 μl) was added onto the slide, and after placing the cover slip, theslide was placed over ice for 10 min. Finally, the cover slips were removed and the slidesimmersed in freshly prepared chilled lysing solution containing 2.5 M NaCl, 100 mMEDTA, and 10 mM Tris (pH10) with 10 % DMSO and 1 % Triton X-100 added just beforeuse. The slides remained in the lysing solution overnight at 4 °C, followed by electrophoresisaccording to the method of Singh et al. ]. The slides were placed in a horizontal gelelectrophoresis tank side by side with agarose ends nearest to the anode. Fresh and chilledelectrophoresis buffer (1 mM Na2 EDTA and 300 mM NaOH, pH>13) was poured into thetank to a level of approximately 2.5 mm above the slides. The slides were left in this solutionfor 25 min to allow DNA unwinding and expression of alkali-labile sites as DNA strandbreaks. Electrophoresis was conducted at 24 V (0.7 V/cm) and a current of 330 mA using apower supply (Electra Comet III from Techno Source India Pvt. Ltd., Mumbai, India) for30 min at 4 °C. All these steps were performed under dimmed light, and the electrophoresistank was covered with black paper to avoid additional DNA damage due to stray light. Afterelectrophoresis, the slides were drained and placed horizontally in a tray. Tris buffer (0.4 M;pH7.5) was added dropwise and left for 5 min to neutralize excess alkali. Neutralizing ofslides was repeated thrice. Each slide was stained with 75 μl EtBr (20 μg/ml) for 5 min anddipped in chilled distilled water to wash off excess EtBr and the cover slip placed over it.
Slides were placed in a dark humidified chamber to prevent drying of the gel. The slideswere scored within 24 h, using an image analysis system (Kinetic Imaging, Liverpool, UK)attached to a fluorescence microscope (Leica, Germany) equipped with appropriate filters
Appl Biochem Biotechnol (2013) 169:1727–1751
(N2.1, excitation wavelength of 515–560 nm and emission wavelength of 590 nm). Themicroscope was connected to a computer through a charge-coupled device camera to transportimages to a software (Komet 5.0) for analysis. The final magnification was ×400. The cometparameters recorded were Olive tail moment (OTM, arbitrary units), tail DNA (percent), andtail length (migration of the DNA from the nucleus, micrometer). Images from 100 cells(50 from each replicate slide) were analyzed as per the in vivo guidelines
Plasma Isolation and Tissue Homogenization
Blood samples were kept at room temperature for 30 min and then centrifuged at 4,000 rpmat 4 °C for 15 min. The supernatant collected as plasma was used for NO, progesterone, andestradiol estimation. Liver, kidney, and ovary were homogenized in 50 mM phosphate buffer(pH7.4) containing protease inhibitor and 0.2 μM PMSF for 30 s using a Polytron homog-enizer. A small portion of the fresh homogenates was first processed for measuring totalROS and the remaining homogenates were centrifuged at 12,000 rpm for 30 min at 4 °C.
The resultant supernatant was used for determining antioxidant enzyme activity bothbiochemically and by native gel. Total protein concentration was measured by Lowry'smethod using BSA as standard.
Biochemical Estimation of Total Nitrite and Nitrate Concentrations in Plasma
Production of nitric oxide (NO), a reactive free radical generally oxidized to NOx (nitrite—NO −
2 /nitrate—NO3 ), is measured by the indirect method of Sastry et al. ] by measuring
total nitrite and nitrate concentrations in plasma using acidic Griess reaction for colordevelopment after the reduction of nitrate with copper–cadmium alloy and deproteinization.
In brief, in 100 μl of sample or standard (KNO3), 400 μl of carbonate buffer followed by asmall amount (0.15 g) of activated copper–cadmium alloy fillings (washed in buffer anddried on a filter paper) was added in tubes and then incubated at room temperature for 1 hwith thorough shaking. The reaction was stopped by the addition of 100 μl of 0.35 Msodium hydroxide (NaOH), followed by 400 μl of 120 mM zinc sulfate (ZnSO4) solutionunder vortex and allowed to stand for 10 min. The tubes were then centrifuged at 8,000 rpmfor 10 min. One-hundred-microliter aliquots of the clear supernatant were transferred intothe wells of a microplate (in quadruplicate), and Griess reagent (50 μl of 1.0 % sulfanilamideprepared in 2.5 % orthophosphoric acid and 50 μl of 0.1 %N-naphthylethylenediamineprepared in distilled water) was added to it with gentle mixing. After 10 min, the absorbancewas measured at 545 nm against a blank (containing the same concentrations of ingredientsbut no biological sample) in a microplate reader (MS5605A, ECIL, Hyderabad, India) usingthe path check option.
Enzyme Immunoassay for Progesterone (P4) and Estradiol (E2)
Steroids in the plasma were measured directly by using commercial ELISA kits (P4-BiotranDiagnostics Inc., Hemet, CA, USA and E2-Labour Diagnostika Nord GmbH & Co. KG,Nordhorn) according to the manufacturer's instructions.
Total ROS Measurement
ROS production was determined in fresh tissue homogenates by using 2′,7′-dichlorodihy-drofluorescein diacetate (DCFH-DA) as a probe, according to Bejma et al. [] with a slight
Appl Biochem Biotechnol (2013) 169:1727–1751
modification. DCFH-DA is a nonpolar compound that, after conversion to a polar derivativeby intracellular esterases, can rapidly react with ROS to form the highly fluorescentcompound dichlorofluorescein. Briefly, the homogenate was diluted in PBS to obtain aconcentration of 25 μg tissue protein/ml. The reaction mixture containing diluted homoge-nate and 10 μl of DCFH-DA (10 μM) was incubated for 15 min at room temperature toallow the DCFH-DA to be incorporated into any membrane-bound vesicles and the diacetategroup cleaved by esterases. After 30 min of further incubation, the conversion of DCFH-DAto the fluorescent product DCF was measured using a spectrofluorimeter with excitation at484 nm and emission at 530 nm. Background fluorescence (conversion of DCFH-DA in theabsence of homogenate) was corrected by the inclusion of parallel blanks.
Biochemical Estimation of Antioxidant Enzymes
Superoxide Dismutase Assay
The method of Das et al. [was used for measuring total SOD activity in liver, kidney,and ovary. The reaction mixture consisted of 100 μl of sample, 150 mM phosphate buffer(pH7.4), 20 mML-methionine, 50 μM EDTA, 1 % Triton X-100, 100 μM riboflavin, andGreiss reagent. The absorbance was taken at 543 nm. The value of SOD was calculatedin terms of units defined as the amount of SOD that inhibits the reduction of nitrobluetetrazolium (NBT) by 50 %. The final results were expressed as unit of SOD permilligram of protein.
Catalase activity in the liver, kidney and ovary was assayed according to Aebi [Briefly,the reaction mixture contained 1.9 ml of 50 mM phosphate buffer (pH7.0) and appropriatedilution of the tissue supernatant to make the volume 2 ml. The reaction was initiated by theaddition of 1 ml of freshly prepared 30 mM H2O2 and the decrease in absorbance wasmeasured at 240 nm for 2–3 min. One unit of catalase represents the decrease of 1 μM ofH2O2 per minute. The molar extinction coefficient for H2O2 is 43.6 M−1cm−1.
Glutathione Peroxidase Assay
GPx activity was assayed in the liver, kidney and ovary as described by Mantha et al. [The reaction mixture (1 ml) contained 50 μl sample, 398 μl of 50 mM phosphate buffer(pH7.0), 2 μl of 1 mM EDTA, 10 μl of 1 mM sodium azide, 500 μl of 0.5 mMNADPH, 40 μl of 0.2 mM GSH and 1 U of glutathione reductase. The reaction mixturewas allowed to equilibrate for 1 min at room temperature. After this, the reaction wasinitiated by the addition of 100 mM H2O2. The absorbance was measured kinetically at340 nm for 3 min. The GPx activity was expressed as micromole of oxidized NADPHoxidized to NADP+ per minute per milligram of protein using an extinction coefficient(6.22 mM−1cm−1) for NADPH.
Assay of Antioxidant Enzymes by Native PAGE
SOD, CAT and GPx activities were also determined by nondenaturing polyacrylamide gelelectrophoresis (native PAGE). SOD assay was performed by using 12 % native gel slab inTris-glycine buffer (pH8.3) at a constant voltage of 100 V. In the case of liver—20 μg,
Appl Biochem Biotechnol (2013) 169:1727–1751
kidney—30 μg and for ovary—30 μg of protein were loaded in each lane. After electro-phoresis, the gel was subjected to substrate-specific staining of SOD bands as described byBeauchamp and Fridovich ]. The staining mixture consisted of 2.5 mM NBT, 28 μMriboflavin and 28 mM TEMED. After 30 min of incubation in the dark, the gel was exposedto a fluorescent light to develop achromatic bands against a dark blue backgroundcorresponding to SOD protein in the gel.
For catalase, a sample containing 20 μg proteins for liver, kidney and ovary was
electrophoresed on 7.5 % native PAGE. Catalase-specific bands were developedaccording to Sun et al. [Briefly, gels were soaked for 10 min in 0.003 %H2O2 and then incubated in a staining mixture consisting of 1 % potassium ferricy-anide and 1 % ferric chloride. Achromatic bands of catalase appeared against a blue–greenbackground.
The active level of GPx was determined by the method of Lin et al. [After
10 % nondenaturing PAGE containing 30 μg protein for liver, 40 μg for kidney and40 μg for ovary, the gels were incubated in a staining mixture composed of 50 mMTris–Cl buffer (pH7.9), 3 mM GSH, 0.004 % H2O2, 1.2 mM NBT and 1.6 mM PMSfor 45 min. Achromatic bands corresponding to GPx activity appeared against aviolet–blue background.
Enzyme activity staining after native PAGE was repeated at least five times and the
intensity of the bands in all the cases was quantitated by gel densitometry using ImageJ v.
1.36 software (NIH, USA).
Statistical Analysis
Statistical analysis was performed after pooling the data of both experiments by usingthe SPSS software. Student's t test was used to compare between the means of thedifferent parameters of the control and experimental groups. The null hypotheses wererejected at p ≤0.05.
Implantation Site
The implantation sites in the 2.45 GHz low-level microwave-irradiated mice wereaffected significantly and exhibited some abnormalities such as unequal/asymmetricaldistribution of embryos in the two uterine horns (Fig. (7)) and reduced inter-embryospaces (Fig. (8)). Further, unlike the sham control, in which a normal number (sixto ten) of pups were born, no pups were born in MW-exposed mice which showedincreased follicular size and some traces of implantation in the uterine horns(Fig. (5, 7, 8)). However, in one mouse, only a single embryo was found inunilateral uterine position (Fig. (6)) which appeared to be bigger in size than those of thecontrol.
It was found that after long-term MW radiation exposure, hemoglobin content (Fig. ) andtotal number of erythrocytes (RBC) (Fig. and leucocytes (WBC) (Fig. increasedsignificantly (p<0.001) in the exposed group of mice as compared to control. Further, a
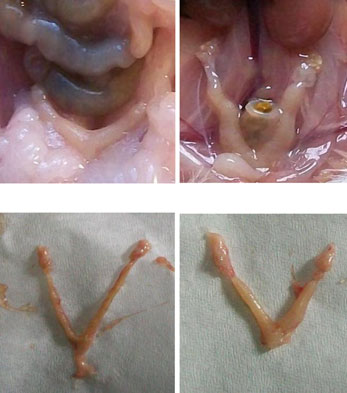
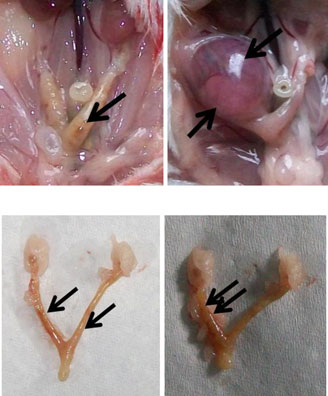
Appl Biochem Biotechnol (2013) 169:1727–1751
MW Exposed
Fig. 2 a Uterine horns of control mice in vivo (1 and 2) and in vitro (3 and 4) after giving birth to pups. bUterine horns of MW-exposed mice in vivo (5 and 6) and in vitro (7 and 8). Unlike the control, nopups were born in the exposed group of mice; however, some traces of implantation as a dark red spot(arrows) can be seen (5, 7, and 8) except in one mouse where only a single embryo (6) was found inunilateral uterine position
significant increase in N/L ratio (p<0.01, Fig. was also observed in the irradiated groupof animals.
We have performed alkaline comet assay to illustrate that prolonged exposure to2.45 GHz microwave radiation caused DNA strand breaks in brain cells of mice.
Microwave-exposed mice showed a significant increase (p<0.001) in OTM (5.3±0.2),tail DNA (16.1 %) and tail length (26 μm) in the brain cells when compared to controlbrain cells (Fig.
Total Nitrite and Nitrate Concentrations
In the microwave-irradiated group of mice, a significant decrease in total nitrite and nitrateconcentrations was observed in plasma when compared to the control group of animals(p<0.05, Fig. This result indirectly shows that the level of NO in female micedecreased after microwave exposure.
Enzyme Immunoassay
We observed an elevation in plasma P4 and E2 concentrations (Fig. , respectively) inthe microwave-irradiated group of mice as compared to the control group. However, asignificant increase was observed only in the E2 level of the microwave-exposed mice(p<0.05), but the increase in P4 concentration was not significant.
Appl Biochem Biotechnol (2013) 169:1727–1751
phocyte 2.5
Fig. 3 Changes in a hemoglobin (Hb) content, b red blood cell (RBC) counts, c white blood cell (WBC)counts, and d neutrophil/lymphocyte (N/L) ratio after 2.45 GHz microwave irradiation in mice. Results areexpressed as mean ± standard deviations (n=12). Significant difference was determined by using Student'st test. **p<0.01 and ***p<0.001, significant difference from thr control group
Total ROS was measured with a DCFH-DA probe to assess the overall oxidative status in liver,kidney and ovary homogenates. An elevated ROS level was observed in the microwave-exposedgroup of mice as compared to control (Fig. ). The microwave-irradiated group showed a significantincrease in fluorescence intensity of the liver (p<0.001), kidney (p<0.01), and ovary (p<0.01).
Antioxidant Enzyme Activity
We have checked whether the nonthermal microwave exposure-induced oxidative stressalters the activity of ROS-scavenging enzymes: SOD, CAT and GPx. Antioxidant enzymeactivities were determined biochemically (spectrophotometrically) as well as by performingnative gel of the liver, kidney and ovary. The results are shown in Table and in Fig.
Superoxide Dismutase Activity
In the exposed group of mice, we observed a significant decrease in the SOD activities of theliver, kidney and ovary as compared to the control (Table , Fig. b(a)). SOD activitywas found to be highly decreased in the liver (p<0.001) of irradiated mice than the kidney(p<0.01) and ovary (p<0.01).
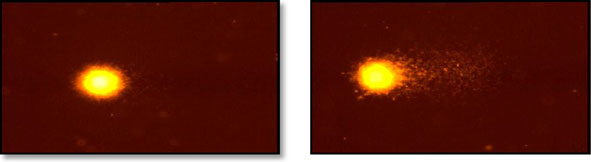
Appl Biochem Biotechnol (2013) 169:1727–1751
OTM (arb. Uni
Tail leng
Fig. 4 DNA damage in the brain cells of control and 2.45 GHzMW-irradiated mice was monitored by cometassay (upper panels, a) and its quantitative measurement (lower panels, b). Results were statistically analyzedby performing Student's t test and are expressed as mean ± standard deviations (n=12). ***p<0.001,significant difference from the control group
Catalase Activity
CAT activities were found to be highly decreased in all the tissues of microwave-irradiatedmice when compared to the control group. This decrease was highly significant, i.e.,p < 0.001 in the liver, kidney and ovary (Table Fig. ), b(b)).
Glutathione Peroxidase Activity
GPx also shows a similar pattern as SOD and CAT. GPx activity was also found to besignificantly decreased (p<0.001) in all the tissues of microwave-exposed mice as comparedto the control mice (Table , Fig. b(c, d)).
Fig. 5 Total nitrite and nitrate
concentrations in the plasma
of MW-exposed mice were
determined to measure NO level
indirectly. A significant decrease
in total nitrite and nitrate
concentrations was observed in
the irradiated group of mice
(n=12). Values are expressed
as mean ± standard deviations.
*p<0.05, significant difference
from the control group
Appl Biochem Biotechnol (2013) 169:1727–1751
Fig. 6 Effect of 2.45 GHz MW
irradiation on circulating a
progesterone and b estradiolconcentrations. No significant
increase was observed in P4concentration while this increase
was significant for E2. Resultswere statistically analyzed by
Student's t test. Values areexpressed as mean ± standard
deviations (n=12).*p<0.05,significant difference from the
a Estradi
uorescence
Fig. 7 Elevation of DCFH-DA fluorescence (measure of ROS) was observed in the liver, kidney and ovary ofMW-irradiated mice as compared to the control group. A highly significant increase was observed in all thetissues. Results are expressed as mean ± standard deviations (n=12). Significant difference was determined byusing Student's t test. **p<0.01 and ***p<0.001, significant difference from the control group
Appl Biochem Biotechnol (2013) 169:1727–1751
Table 1 Comparative statistical analysis of changes in antioxidant enzyme activities: superoxide dismutase(SOD), catalase (CAT) and glutathione peroxidase (GPx) in 2.45 GHz microwave-irradiated mice. Values areexpressed as mean ± standard deviation (n=12)
SOD (U/mg protein)
CAT (μmol/min/mg protein)
GPx (μmol/min/mg protein)
The present study clearly demonstrates for the first time that low-level 2.45 GHz MWradiation is able to affect implantation/pregnancy via inducing oxidative stress. Our studyelucidated that in MW-exposed mice, implantation sites were affected significantly whencompared to control. This is consistent with our previous study and other investigations onmice exposed to 2.45 GHz (CW) at a power density of 30 mW/cm2 have shown decreasedimplantation sites per litter and reduction in fetal weight during 1–6 days of gestation.
Exposure of mice at the same power density but 6–15 days of gestation resulted in a slightbut significant increase in the percentage of malformed fetuses, predominantly with cleftpalate [We also observed some abnormalities such as unequal/asymmetrical distributionof embryos in the two uterine horns and reduced inter-embryo spaces in the exposed groupof mice. No pups were born in the exposed group unlike the control, although some traces ofembryos were observed in the uterine horns. However, in one mouse, only a single embryoof abnormally increased size and deformed was found in unilateral uterine position. Thiscould be due to resorption of embryo during early pregnancy as a consequence of MWradiation-induced oxidative stress which also exerts adverse effects on birth outcome. It wasfound that exposure to an electromagnetic field during pregnancy was linked to an increasedrisk of miscarriage , ]. Pregnant women and children are exposed to this low-level MWradiation (especially by microwaves, mobile phones, and Wi-Fi signals) in the same degreeas the general public. A study on human females also suggests that pregnant physiothera-pists, who are exposed to microwaves and shortwave as a result of their occupation, had anincreased risk of miscarriage. A total of 1,753 pregnancies involving first trimester miscar-riages were matched to 1,753 control pregnancies. This revealed a 7 % but nonsignificantrise in miscarriages associated with shortwave exposure and a significant 28 % increase infirst trimester miscarriages for those exposed to microwaves, including a highly significantdose–response relationship [However, our results contradict with the reports ofSambucci et al. and Jensh et al. , which had shown no remarkable effect of2.45 GHz MW radiation exposure on pregnancy or the development of preimplantationembryo. We can only speculate on the explanation of our results that the resorption of theembryo might have occurred due to MW radiation-induced oxidative stress.
Oxidative stress is a pronounced prooxidant state, caused by the excessive production of
free radicals or from the weakening of the antioxidant defense system. Our experimental dataclearly show that exposure to MW radiation led to the generation of ROS. Our resultssupport the earlier study which suggests that extremely low frequency magnetic field as apotent activator of macrophages produces a large amount of ROS []. An imbalance
Appl Biochem Biotechnol (2013) 169:1727–1751
Fig. 8 a Decreases in antioxidant enzyme activities of a SOD (upper panel), b CAT (middle panel), and c
GPx (lower panel) were observed in the liver, kidney, and ovary of female mice after 2.45 GHz MWirradiation. Data were statistically analyzed by Student's t test. Values are mean ± standard deviations(n=12). **p<0.01 and ***p<0.001, significant difference from the control group. b Changes in antioxidantenzyme activities of SOD (a), CAT (b), and GPx (c, d) in the liver, kidney, and ovary of 2.45 GHzMW-irradiatedmice were demonstrated by native gel and enzyme activity staining. The intensity of bands in all the cases wasquantitated by gel densitometry using ImageJ v. 1.36 software (NIH). Significant difference was determined byusing Student's t test. Values are expressed as mean ± standard deviations. **p<0.01 and ***p<0.001,significant difference from the control group
between the production and manifestation of ROS, i.e., extensive production of peroxidesand free radicals, causes toxic effects and damages all components of a cell includingproteins, lipids and DNA. We also observed a highly significant DNA damage in the braincells of mice after low-level MW exposure which is supported by our previous study andalso by Lai and Singh [This could be due to the elevated ROS load, providingnucleophilic SN2 attack on the sugar moiety of the nucleotide residues producing apurinicor apyrimidinic sites, causing single- or double-stranded DNA breaks. ROS speciallyhydroxyl ions (•OH) generated close to DNA attack the base pair and may cause mutation[Increased DNA damage consequently affects the embryonic development and couldincrease the chances of abnormal embryo or may also result in resorption. We have alsoobserved an alteration in total protein content of liver, kidney and ovary but this alterationwas insignificant.
ROS plays a role in the modulation of an entire spectrum of physiological reproductive
functions such as oocyte maturation, ovarian steroidogenesis, corpus luteal functions,luteolysis, fertilization, embryo development and pregnancy [To ensure physiologicallevels of ROS, oocytes and granulosa cells in all follicular stages as well as follicular fluidare well endowed with the major antioxidant and detoxifying enzymes [During theestrous cycle, ovarian follicles, corpus luteum (CL) and the uterus exhibit rapid cellularproliferation, growth and development to ensure a hormonal environment suitable for earlyembryonic development and the establishment of pregnancy. Estradiol produced by thedeveloping ovarian follicles interacts with progesterone produced by the CL to induceuterine receptivity for embryo implantation. Normally, during and just after pregnancy, thelevel of estradiol remains low due to the inhibitory effect of progesterone and prolactin. Butin case of the MW-irradiated group of animals, we found a significant increase in estradiollevel. We also observed an insignificant increase in progesterone level which might be due tothe presence of functional corpus lutea in the ovary of MW-exposed mice. This suggests thepossible effect of MW radiation-induced oxidative stress as a consequence of elevated ROSon steroidogenesis. We also suggest that the elevated estradiol level affects the secretion ofprogesterone during the gestation period, and thus, it could not maintain the pregnancy afterimplantation. ROS is also implicated in the induction of apoptosis ] and cellular senes-cence It may affect the dynamic processes of gamete maturation, its transportationthrough the reproductive tract, and fertilization and thus, consequently affect the integrity,viability, and function of gametes and embryos. Further, the subsequent development andimplantation of the preimplantation embryo get affected by ROS. We observed a significantincrease in hemoglobin content as well as RBC and WBC counts in female mice exposed tolow-level MW radiation. We also found a significant increase in N/L ratio which is anindicator of a hematologic response to inflammatory stimuli and stress. All these changesmight have occurred in response to increased ROS production after weakening of theantioxidant defense system. It is also well-established that an oxidative stress condition affectsthe whole body physiology of the animal including its blood profile and blood cell number.
Appl Biochem Biotechnol (2013) 169:1727–1751
protei
g 0.12
o 0.06
(µm 0.04
in/m 0.015
(µm 0.005


Appl Biochem Biotechnol (2013) 169:1727–1751
1. Superoxide dismutase activity
Cont. Exp.
Cont. Exp.
2. Catalase activity
Cont. Exp. Cont. Exp. Cont.
Kidney Ovary
Fig. 8 (continued)
Any alteration in ROS level directly indicates changes in antioxidant enzyme activities;
therefore, we next examined ROS-scavenging enzymes SOD, CAT and GPx activitiesresponsible for inducing oxidative stress. MW-irradiated mice show a significant decreasein the antioxidant enzyme activities in the liver, kidney and ovary. This decreased antiox-idant activity results in response to elevated ROS level, which causes oxidative damage,although we found that the liver is much more affected than the kidney and ovary which may
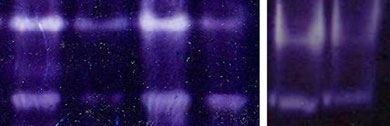
Appl Biochem Biotechnol (2013) 169:1727–1751
3. Glutathione peroxidase activity
Cont. Exp.
Cont. Exp. Cont. Exp.
Fig. 8 (continued)
be quite possible because the liver is the main detoxifying organ that metabolized MW-induced toxicity, i.e., ROS. Further, we also observed that CAT activity consistently de-creased in all the tissues than SOD and GPx. CAT plays a major role in protecting the cellagainst oxidative damage by catalyzing the decomposition of H2O2 to water and molecularoxygen. Decreased CAT activity may compromise the overall antioxidant enzyme defensesystem. It is also well known that GPx is not an efficient H2O2 decomposer as compared toCAT, and hence, a high level of H2O2 is observed in CAT-depleted cells than GPx-sufficientcells. Therefore, the compromised CAT activities may significantly impair the capacity of theantioxidant enzyme defense system. CAT and GPx are well-known H2O2 scavengers whichserve to protect cells from the toxic effects of H2O2. Low-level MW exposure decreased
Appl Biochem Biotechnol (2013) 169:1727–1751
SOD activity significantly which could enhance the production of O •−
dismutase by SOD undergoes nonenzymatic reaction to form H2O2, a precursor of the moretoxic hydroxyl radical. Since the activities of these enzymes were significantly decreased inirradiated mice, the level of H2O2 increased. This increased H2O2 production may beresponsible for elevated embryo fragmentation, suggesting that ROS may be implicated inthe induction of apoptosis in embryos [].
We also found a significant decrease in NO level in the exposed group of mice. A
decrease in NO level may result in cell toxicity by increasing free radical load since it canalso act as a free radical scavenger and inactivate O •−
, thereby preventing cell toxicity [
NO causes vasoconstriction within the vasculature which may result in hypertension.
Normally, low oxygen tension is required to enhance embryo development to the blastocyststage and to increase their total cell counts by reducing the proportion of apoptotic cells ,]. This enhanced embryo development is thought to be responsible for improved embryometabolism []. Reduced NO level also affects the pregnancy/implantation by affectingthe embryonic growth and development. However, it has been reported that during normalpregnancy, NO production increases with advancing gestation which returns to its normallevel within 12 weeks after delivery ]. The increased nitric oxide synthesis may bepartially responsible for regulating the vascular adaptation of pregnancy while reductionof nitric oxide production in rats results in a significant intrauterine growth retardation [NO regulates the uterine and fetoplacental blood flow and could be involved in uterinequiescence prior to parturition.
The results of our present study clearly demonstrate that MW irradiation results in the
accumulation of havoc-free radicals in different organs and thus induced oxidative stresswhich is in accordance with the earlier published studies [, Elevated free radicals notonly disturb the normal physiology of the body but also exert an adverse effect on thepregnancy or implantation in female mice. There are several studies which demonstrate thatthe imbalance in free radicals and antioxidant enzymes responsible for causing oxidativestress influences the timing and maintenance of viable pregnancy. A review by Gray andBecker [] also suggests that the delayed conception and the early pregnancy loss mayshare a common etiology, possibly through events or exposures prior to or during implan-tation and embryogenesis. Our results demonstrate that MW irradiation is responsible forgenerating extensive free radical load due to a significant reduction in antioxidant activitiesand NO production which could possibly cause the aging and exhaustion of the ovary andincreased the contractile activity of the uterus. The accumulation of damage exerted byincreased ROS levels is claimed to be involved in ovarian aging [] and hormone alteration.
The inadequate hormone production has been proposed as a cause of embryonic losses inhumans [and ruminants ROS is among the most important physiological inducersof cellular injury associated with aging []. Oocyte aging and deteriorating oocyte qualityknown to occur with advancing age and many pathological conditions contribute signifi-cantly to reproductive failure. Miscarriage during the establishment of cellular and biochem-ical interactions between the uterine endometrium and the developing post-implantingconceptus is associated with increased oxidative stress ].
Therefore, on the basis of our observations, we propose that the resorption of the embryo/
fetus in MW-irradiated mice might have occurred due to altered estradiol and progesteronelevels, increased cellular fragmentation, and gross abnormal morphology which may resultfrom oxidative damage to cytoskeletal fibers. This could be due to increased ROS loadcaused by the reduction in NO level and antioxidant enzyme activities, responsible foroocyte aging, hormonal changes, DNA damage, and protein expression. There is evidencesupporting the concept that oxidative damage to cytoskeletal fibers may result in abnormal
Appl Biochem Biotechnol (2013) 169:1727–1751
embryo morphology. In contrast to the surface of vital blastomeres, which is organized intoshort, regular microvilli, the surface of cellular fragments exhibits irregularly shaped blebsand protrusions ]. Cytoskeletal alterations associated with the formation of surface blebsare characteristic signals of oxidative cell injury. It is also well-established that the elevatedROS levels generate damage to cells/embryos through increased lipid peroxidation ]and protein oxidation and induce DNA strand breaks [
In spite of the number of reports indicating radiation-induced oxidative stress and its
effects on implantation/pregnancy, the precise mechanism on how MW radiation inducedthese effects is still unknown. However, we suggest that all these effects of MW radiation atlow SAR value might have been caused due to resonant absorption of microwave by theanimal body. Also, there is a possibility of molecular resonance absorption of energy bygenetic material. The energy can be absorbed over time involving many photons. Therefore,the molecule can absorb the energy of not just one photon but many photons. This energymay be smaller compared with the total thermal energy already present in the molecule,but more than enough to affect its performance, especially if it is all in one oscillationmode
On the basis of our experimental findings, we conclude that 2.45 GHz MW radiation,
a nonthermal electromagnetic radiation, acts as a strong environmental stressor responsi-ble for inducing oxidative stress. It increases ROS load, hemoglobin, RBC and WBCcount, N/L ratio as well as P4 and E2 concentrations. Decreased NO and antioxidantenzyme activity (SOD, CAT and GPx) observed in the 2.45 GHz low-level MW-irradiated mice may be correlated with increased ROS which adversely affects theimplantation or pregnancy in mice. Enhancement of ROS, altered antioxidant activitiesand sex steroid level responsible for oocyte aging and deteriorated oocyte/embryo lead toimplantation failure and/or resorption. Further, MW radiation-induced oxidative stressmay also affect the timing of implantation, maintenance of viable pregnancy, and prenataldevelopment. However, further studies are required to understand the exact mechanismand site of action of low-level MW radiation and its effect on implantation/pregnancy viaoxidative damage.
Acknowledgments This work was funded by a research grant (5/10/FR/13/2010-RHN) from the IndianCouncil of Medical Research, New Delhi, India to CMC.
1. Stuchley, M. A. (1988). Biological effects of radiofrequency fields. In: M.H. Repacholi (Ed.) Non-ionizing
radiations, physical characterization, biological effects and health hazard assessment. Proceedings for theInternational Non-Ionizing Radiation workshop, Melbourne, 197–217.
2. Lai, H., Carino, M. A., Horita, A., & Guy, A. W. (1993). Effects of a 60 Hz magnetic field on central
cholinergic system of the rat. Bioelectromagnetics, 14, 5–15.
3. Lokhmatova, S. A. (1994). The effect of low-intensity prolonged impulse electromagnetic irradiation in
the UHF range on the testis and the appendages of the testis in rats. Radiation Biology and Radioecology,34, 279–285.
4. Akdag, M. Z., Celik, M. S., Ketani, A., Nergiz, Y., Deniz, M., & Dsadag, S. (1999). Effect of chronic low
intensity microwave radiation on sperm count, sperm morphology and testicular and epididymal tissues ofrat. Electro- and Magnetobiology, 18, 133–145.
5. Hardell, L., Mild, K. H., & Carlberg, M. (2003). Further aspects on cellular and cordless telephones and
brain tumours. International Journal of Oncology, 22, 399–407.
6. Heynick, L. N., Johnston, S. A., Patrick, A., & Mason, P. A. (2003). Radio frequency electromagnetic
fields: cancer, mutagenesis, and genotoxicity. Bioelectromagnetics, 24, s74–s100.
Appl Biochem Biotechnol (2013) 169:1727–1751
7. Paulraj, R., & Behari, J. (2004). Radiofrequency radiation effect on protein kinase C activity in rats' brain.
Mutation Research, 545, 127–130.
8. Agarwal, A., Deepinder, F., Sharma, R. K., Ranga, G., & Li, J. (2008). Effect of cell phone usage
on semen analysis in men attending infertility clinic: an observational study. Fertility and Sterility,89, 124–128.
9. Cleveland, R. F., & Ulcek, J. L. (1999). Questions and answers about biological effects and potential
hazards of radiofrequency electromagnetic fields. OET Bulletin, 56, 1–36.
10. Fukui, Y., Hoshino, K., Inouye, M., & Kameyama, Y. (1992). Effects of hyperthermia induced by the
microwave irradiation on the brain development in mice. Journal of Radiation Research, 33, 1–10.
11. Paulraj, R., & Behari, J. (2006). Single strand DNA breaks in rat brain cells exposed to microwave
radiation. Mutation Research/Fundamental and Molecular Mutagenesis, 596, 76–80.
12. Kesari, K. K., Behari, J., & Kumar, S. (2010). Mutagenic response of 2.45 GHz radiation exposure on rat
brain. International Journal of Radiation Biology, 86(4), 334–343.
13. Saunders, R. D., & Kowalczuk, C. I. (1981). Effects of 2.45 GHz microwave radiation and heat on mouse
spermatogenic epithelium. International Journal of Radiation Biology, 40(6), 623–632.
14. Kim, J. Y., Kim, H. T., Moon, K. H., & Shin, H. J. (2007). Long-term exposure of rats to a 2.45 GHz
electromagnetic field: effects on reproductive function. Korean Journal of Urology, 48(12), 1308–1314.
15. Kesari, K. K., & Behari, J. (2010). Effects of microwave at 2.45 GHz radiations on reproductive system of
male rats. Toxicological and Environmental Chemistry, 92(6), 1135–1147.
16. Galvin, M. J., MacNichols, G. L., & McRee, D. I. (1984). Effect of 2450 MHz microwave radiation on
haematopoiesis of pregnant mice. Radiation Research, 100, 412–417.
17. Trosic, I., & Busljeta, I. (2005). Frequency of micronucleated erythrocytes in rat bone marrow exposed to
2.4 5GHz radiation. Physica Scripta, T118, 168–170.
18. World Health Organization (2011). World Health Organization/International Agency for Research on
Cancer classifies radiofrequency electromagnetic fields as possibly carcinogenic to humans. 31May.ScienceDaily.
19. Luria, R., Eliyahu, I., Hareuveny, R., Margaliot, M., & Meiran, N. (2009). Cognitive effects of radiation
emitted by cellular phones: the influence of exposure side and time. Bioelectromagnetics, 3, 198–204.
20. Hardell, L., Carlberg, M., & Hansson, M. K. (2009). Epidemiological evidence for an association between
use of wireless phones and tumor diseases. Pathophysiology, 16, 113–122.
21. Hardell, L., Carlberg, M., & Hansson, M. K. (2011). Pooled analysis of case–control studies on malignant
brain tumours and the use of mobile and cordless phones including living and deceased subjects.
International Journal of Oncology, 38(5), 1465–1474.
22. Pryor, W. A., Houk, K. N., Foote, C. S., Fukuto, J. M., Ignarro, L. J., Squadrito, G. H., et al. (2006). Free
radical biology and medicine: it's a gas, man! American Journal of Physiology - Regulatory, Integrativeand Comparative Physiology, 291, R491–R511.
23. Eriksson, U. J. (1999). Oxidative DNA damage and embryo development. Nature Medicine, 5, 715.
24. Baker, M. A., & Aitken, R. J. (2005). Reactive oxygen species in spermatozoa: methods for monitoring
and significance for the origins of genetic disease and infertility. Reproductive Biology andEndocrinology, 3, 67.
25. Palacio, J. R., Iborra, A., Ulcova-Gallova, Z., Badia, R., & Martinez, P. (2006). The presence of antibodies
to oxidative modified proteins in serum from polycystic ovary syndrome patients. Clinical andExperimental Immunology, 144, 217–222.
26. Liu, H., Zhang, C., & Zeng, W. (2006). Estrogenic and antioxidant effects of a phytoestrogen daidzein on
ovarian germ cells in embryonic chickens. Domestic Animal Endocrinology, 31, 258–268.
27. Takahashi, T., Takahashi, E., Igarashi, H., Tezuka, N., & Kurachi, H. (2003). Impact of oxidative stress in
aged mouse oocytes on calcium oscillations at fertilization. Molecular Reproduction and Development,66, 143–152.
28. Kodaman, P. H., & Behrman, H. R. (2001). Endocrine-regulated and protein kinase C-dependent
generation of superoxide by rat preovulatory follicles. Endocrinology, 142, 687–693.
29. Dincer, Y., Akcay, T., Erdem, T., Saygili, I. E., & Gundogdu, S. (2005). DNA damage, DNA susceptibility
to oxidation and glutathione level in women with polycystic ovary syndrome. Scandinavian Journal ofClinical and Laboratory Investigation, 65, 721–728.
30. Guerin, P. E., Mouatassim, S., & Menezo, Y. (2001). Oxidative stress and protection against reactive
oxygen species in the pre-implantation embryo and its surroundings. Human Reproduction Update,7, 175–189.
31. Conrad, K. P., Joffe, G. M., Kruszyna, H., et al. (1993). Identification of increased nitric oxide
biosynthesis during pregnancy in rats. The FASEB Journal, 7, 566–571.
32. Beckman, J. S., & Crow, J. P. (1993). Pathological implications of nitric oxide, superoxide and
peroxynitrite formation. Biochemical Society Transactions, 21, 330–334.
Appl Biochem Biotechnol (2013) 169:1727–1751
33. Davies, M. G., Fulton, G. J., & Hagen, P. O. (1995). Clinical biology of nitric oxide. British Journal of
Surgery, 82, 1598–1610.
34. Akaike, T., Suga, M., & Maeda, H. (1998). Free radicals in viral pathogenesis: molecular mechanisms
involving superoxide and NO. Proceedings of the Society for Experimental Biology and Medicine,217, 64–73.
35. Turko, I. V., & Murad, F. (2002). Protein nitration in cardiovascular diseases. Pharmacological Reviews,
54, 619–634.
36. Kelly, R. A., Balligand, J. L., & Smith, T. N. (1996). Nitric oxide and cardiac function. Circulation
Research, 79, 363–380.
37. Cameron, I. T., & Campbell, S. (1998). Nitric oxide in the endometrium. Human Reproduction Update,
4, 565–569.
38. Rollwitz, J., Lupke, M., & Simko, M. (2004). Fifty-hertz magnetic fields induce free radical formation
in mouse bone marrow-derived promonocytes and macrophages [J]. Biochimica et Biophysica Acta,1674(3), 231–238.
39. Ciejka, E., Kleniewska, P., Skibska, B., & Goraca, A. (2011). Effects of extremely low frequency
magnetic field on oxidative balance in brain of rats. Journal of Physiology and Pharmacology,62(6), 657–661.
40. Rugh, R., Ginns, E. I., Ho, H. S., & Leachw, M. (1975). Responses of the mouse to microwave radiation
during estrous cycle and pregnancy. Radiation Research, 62, 225–241.
41. Nawrot, P. S., McRee, D. I., & Staples, R. E. (1981). Effects of 2.45 GHz CW microwave radiation on
embryofetal development in mice. Teratology, 24(3), 303–314.
42. Nakamura, H., Matsuzaki, I., Hatta, K., Nobukuni, Y., Kambayashi, Y., & Ogino, K. (2003). Nonthermal
effects of mobile-phone frequency microwaves on uteroplacental functions in pregnant rats. ReproductiveToxicology, 17, 321–326.
43. Inouye, M., Galvin, M. J., McRee, D. I., & Matsumoto, N. (1982). Lack of effect of 2.45-GHz microwave
radiation on the development of preimplantation embryos of mice. Bioelectromagnetics, 3, 275–283.
44. Jensh, R. P., Weinberg, I., & Brent, R. L. (1983). An evaluation of the teratogenic potential of protracted
exposure of pregnant rats to 2450–MHz microwave radiation: I. Morphologic analysis at term. Journal ofToxicology and Environmental Health, 11, 23–35.
45. Sambucci, M., Laudisi, F., Nasta, F., Pinto, R., Lodato, R., Altavista, P., et al. (2010). Prenatal exposure to
non-ionizing radiation: effects of WiFi signals on pregnancy outcome, peripheral B-cell compartment andantibody production. Radiation Research, 174, 732–740.
46. Singh, V. P., Singh, P., Shukla, R. K., Dhawan, A., Gangwar, R. K., Singh, S. P., et al. (2009). 2.45 GHz
low level CW microwave radiation affects embryo implantation sites and single strand DNA damage inbrain cell of mice, Mus musculus. In: Proceedings of 2009 International Conference on Emerging Trends inElectronic and Photonic Devices & Systems (ELECTRO-2009) from IEEE Explore, 22–24 pp 379–382.
47. Chaturvedi, C. M., Singh, V. P., Singh, P., Basu, P., Singaravel, M., Shukla, R. K., et al. (2011). 2.45 GHz
(CW) Microwave irradiation alters circadian organization, spatial memory, DNA structure in the braincells and blood cell counts of male mice, Mus musculus. Progress in Electromagnetics Research B,29, 23–42.
48. Hartmann, A., Agurell, E., Beevers, C., Brendler-Schwaab, S., Burlinson, B., Clay, P., et al. (2003).
Recommendations for conducting the in vivo alkaline Comet assay. Mutagenesis, 18, 45–51.
49. Patel, S., Pandey, A. K., Bajpayee, M., Parmar, D., & Dhawan, A. (2006). Cypermethrin-induced DNA
damage in organs and tissues of the mouse: evidence from the comet assay. Mutation Research, 607, 176–183.
50. Singh, N. P., McCoy, M. T., Tice, R. R., & Schneider, E. L. (1988). A simple technique for quantitation of
low levels of DNA damage in individual cells. Experimental Cell Research, 175, 184–191.
51. Sastry, K. V., Moudgal, R. P., Mohan, J., Tyagi, J. S., & Rao, G. S. (2002). Spectrophotometric
determination of serum nitrite and nitrate by copper. Analytical Biochemistry, 306, 79–82.
52. Bejma, J., Ramires, P., & Ji, L. L. (2000). Free radical generation and oxidative stress with ageing and
exercise: differential effects in the myocardium and liver. Acta Physiologica Scandinavica, 169, 343–351.
53. Das, K., Samanta, L., & Chainy, G. B. N. (2000). A modified spectrophotometric assay of superoxide
dismutase using nitrite formation by superoxide radicals. Indian Journal of Biochemistry & Biophysics,37, 201–204.
54. Aebi, H. (1984). Catalase in vitro. Methods in Enzymology, 105, 121.
55. Mantha, S. V., Prasad, M., Kalra, J., & Prasad, K. (1993). Atherosclerosis, 101, 135–144.
56. Beauchamp, C., & Fridovich, I. (1971). Superoxide dismutase: improved assays and an assay applicable
to acrylamide gels. Analytical Biochemistry, 44, 276–287.
57. Sun, Y., Elwell, J. H., & Oberley, L. W. (1988). A simultaneous visualization of the antioxidant enzymes
glutathione peroxidase and catalase on polyacrylamide gels. Free Radical Research Communications,5, 67–75.
Appl Biochem Biotechnol (2013) 169:1727–1751
58. Lin, C. L., Chen, H. J., & Hou, W. C. (2002). Activity staining of glutathione peroxidase after
electrophoresis on native and sodium dodecylsulfate polyacrylamide gels. Electrophoresis, 23, 513–516.
59. Lee, G. M., Neutra, R. R., Hristova, L., Yost, M., & Hiatt, R. A. (2002). A nested case–control study of
residential and personal magnetic field measures and miscarriages. Epidemiology, 13(1), 21–31.
60. Li, D. K., Odouli, R., Wi, S., Janevic, T., Golditch, I., Bracken, T. D., et al. (2002). A population-based
prospective cohort study of personal exposure to magnetic fields during pregnancy and the risk ofmiscarriage. Epidemiology, 13(1), 9–20.
61. Ouellet-Hellstrom, R., & Stewart, W. F. (1993). Miscarriages among female physical therapists who
report using radio- and microwave-frequency electromagnetic radiation. American Journal of Epidemiology,138(10), 775–786.
62. Frahm, J., Mattsson, M. O., & Simko, M. (2010). Exposure to ELF magnetic fields modulates redox
related protein expression in mouse macrophages. Toxicology Letters, 192, 330–336.
63. Lai, H., & Singh, N. P. (1996). Single- and double-strand DNA breaks in rat brain cells after acute
exposure to radiofrequency electromagnetic radiation. International Journal of Radiation Biology,69, 513–521.
64. Regoli, F., Gorbi, S., Machelle, N., Tedesco, S., Benedetti, M., Bocchetti, R., et al. (2005). Prooxidant
effects of extremely low frequency electromagnetic fields in the land snail Helix aspersa. Free RadicalBiology & Medicine, 39, 1620.
65. Agarwal, A., Gupta, S., & Sharma, R. K. (2005). Role of oxidative stress in female reproduction.
Reproductive Biology and Endocrinology, 3, 28.
66. Mouatassim, S. E., Guerin, P., & Menezo, Y. (1999). Oxidative stress and protection against reactive
oxygen species in the pre-implantation embryo and its surroundings. Molecular Human Reproduction,5, 720–725.
67. Carbone, M. C., Tatone, C., DelleMonache, S., Marci, R., Caserta, D., Colonna, R., et al. (2003).
Antioxidant enzymatic defences in human follicular fluid: characterization and age-dependent changes.
Molecular Human Reproduction, 9, 639–643.
68. Stone, J. R., & Yang, S. (2006). Hydrogen peroxide: a signaling messenger. Antioxidants & Redox
Signaling, 8, 243–270.
69. Passos, J. F., Saretzki, G., Ahmed, S., Nelson, G., Richter, T., Peters, H., et al. (2007). Mitochondrial
dysfunction accounts for the stochastic heterogeneity in telomere-dependent senescence. PLoSBiology, 5, 110.
70. Yang, H. W., Hwang, K. J., Kwon, H. C., Kim, H. S., Choi, K. W., & Oh, K. S. (1998). Detection of
reactive oxygen species (ROS) and apoptosis in human fragmented embryos. Human Reproduction,13, 998–1002.
71. Cook, J. P., & Tsao, P. S. (1993). Cytoprotective effects of nitric oxide. Circulation, 88, 2451–2454.
72. Gardner, D. K., & Lane, M. (1996). Alleviation of the ‘2-cell block' and development to the blastocyst of
CF1 mouse embryos: role of amino acids, EDTA and physical parameters. Human Reproduction,11, 2703–2712.
73. Van Soom, A., Yuan, Y. Q., Peelman, L. J., de Matos, D. G., Dewulf, J., Laevens, H., et al. (2002).
Prevalence of apoptosis and inner cell allocation in bovine embryos cultured under different oxygentensions with or without cysteine addition. Theriogenology, 57, 1453–1465.
74. Khurana, N. K., & Wales, R. G. (1989). Effects of oxygen concentration on the metabolism of [U-14C]
glucose by mouse morulae and early blastocysts in vitro. Reproduction, Fertility, and Development,1, 99–106.
75. Du, Z. F., & Wales, R. G. (1993). Glycolysis and glucose oxidation by the sheep conceptus at different
oxygen concentrations. Reproduction, Fertility, and Development, 5, 383–393.
76. Choi, J. W., Im, M. W., Pai, S. H. (2002). Nitric oxide production increases during normal pregnancy and
decreases in preeclampsia. Annals of Clinical & Laboratory Science, 32(3).
77. Yang, D., Lang, U., Greenberg, S. G., Myatt, L., & Clark, K. E. (1996). Elevation of nitrate levels in
pregnant ewes and their fetuses. American Journal of Obstetrics and Gynecology, 174, 573–577.
78. Gray, R. H., & Becker, S. (2000). Selected topics in the epidemiology of reproductive outcomes.
Epidemiologic Reviews, 22, 71–75.
79. Tarin, J. J. (1995). Aetiology of age-associated aneuploidy: a mechanism based on the ‘free radical theory
of ageing'. Molecular Human Reproduction, 10, 1563–1565.
80. Porter, T. F., & Scott, J. R. (2005). Evidence-based care of recurrent miscarriage. Best Practice &
Research. Clinical Obstetrics & Gynaecology, 19, 85–101.
81. Diskin, M. G., & Morris, D. G. (2008). Embryonic and early foetal losses in cattle and other ruminants.
Reproduction in Domestic Animals, 43(Suppl. 2), 260–267.
82. Harman, D. (1988). Free radical theory of aging: an update: increasing the functional life span. Annals of
the New York Academy of Sciences, 1067, 10–21.
Appl Biochem Biotechnol (2013) 169:1727–1751
83. Jenkins, C., Wilson, R., Roberts, J., Miller, H., McKillop, J. H., & Walker, J. J. (2000). Antioxidants: their
role in pregnancy and miscarriage. Antioxidants & Redox Signaling, 2, 623ox.
84. Alikani, M., Dale, B. (1995). Should fragmenting blastomeres stay together? Alpha, September 1–2.
85. Nasr-Esfahani, M. H., Aitken, J. R., & Johnson, M. H. (1990). Hydrogen peroxide levels in mouse
oocytes and early cleavage stage embryos developed in vitro or in vivo. Development, 109, 501–507.
86. Nasr-Esfahani, M. H., & Johnson, M. H. (1992). Quantitative analysis of cellular glutathione in early
preimplantation mouse embryos developing in vivo and in vitro. Human Reproduction, 7, 1281–1290.
87. Orsi, N. M., & Leese, H. J. (2001). Protection against reactive oxygen species during mouse preimplan-
tation embryo development: role of EDTA, oxygen tension, catalase, superoxide dismutase and pyruvate.
Molecular Reproduction and Development, 59, 44–53.
88. Fröhlich, H. (1981). The biological effects of microwaves and related questions. Advances in Electronics
and Electron Physics, 53, 85–152.
89. Lerner, E. J. (Contributing Editor) (1984). Biological effects of electromagnetic fields. IEEE spectrum,
Source: http://wifiinschools.org.uk/resources/Shahin+et+al+2013.pdf
Psilocybin Investigator's Brochure March-April 2007 Psilocybin: Investigator's Brochure Table of Contents 1. Drug Substance and Formulation. 3 2. Pharmacological and toxicological effects . 3 Psilocybin and Psilocin Actions on Neurotransmitter Systems . 3 Overview. 3 Psilocybin or psilocin and 5HT2A and 5HT2C Receptors . 4 Psilocybin or Psilocin and other Serotonin Receptors . 4 Psilocybin or Psilocin and other Receptor Systems. 5 Human Neuropharmacological Studies . 6 Psilocybin and Gene Expression. 6
Diabetic foot ulcers – prevention and treatment A Coloplast quick guide Biatain® – the simple choice Diabetic foot ulcers have a considerable negative impact on The diabetic foot – a clinical challenge . 5 patients' lives, and are highly susceptible to infection that al too Pathway to clinical care and clinical evidence . 6 often leads to amputation. It is essential that diabetic foot ulcers