The relationship between riverine lithium isotope composition and silicate weathering rates in iceland
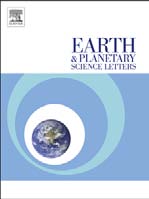
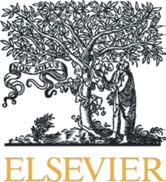
Earth and Planetary Science Letters 287 (2009) 434–441
Contents lists available at
Earth and Planetary Science Letters
The relationship between riverine lithium isotope composition and silicateweathering rates in Iceland
N. Vigier , S.R. Gislason K.W. Burton , R. Millot , F. Mokadem a CRPG-CNRS, Nancy-Université, 15 rue ND des Pauvres, 54501 Vandoeuvre les Nancy Cedex, Franceb Univ. of Iceland, Icelandc The Open University, Milton Keynes, UKd BRGM, Metrology, Monitoring, Analysis Division, 3 Av. Claude Guillemin, BP 6009, 45060 Orleans Cedex 2, France
This study presents lithium isotope and elemental data for the dissolved phase and suspended and bedload
Received 23 July 2009
sediments of the major Icelandic rivers. For the dissolved phase, δ7Li values range between 10.1‰ and
Accepted 20 August 2009
23.8‰, while river sediments display lower and much more homogeneous values (δ7Li = 3.1‰–4.8‰), close
Available online 9 September 2009
to the composition of unweathered Mid-Ocean Ridge Basalt (MORB). High δ7Li values are associated with
Editor: M.L. Delaney
high K/Li, Na/Li and Mg/Li ratios, in waters draining mainly old and weathered basalt catchments, whereaslow δ7Li rivers are located in younger parts of the island. Simple mixing between precipitation, Li-rich
hydrothermal springs and basalt weathering is unable to explain the entire range of δ7Li values. Instead, a
simple model of Li uptake by secondary minerals, associated with clay–water Li isotope fractionation (Δ7Li
ranging from −1‰ to -7.5‰) can explain both water and sediment δ7Li values. A negative correlation is
silicate weathering
observed between basalt chemical erosion rates and δ7Li measured in Icelandic rivers, and an empirical law
is inferred. Comparison with literature data suggests that this relationship may be applicable at a more global
scale, and, if confirmed, could be of particular use for estimating the evolution of continental weatheringpreserved in marine sedimentary records. However, more data are now needed for rivers draining silicatestypical of the continental crust, in order to refine large scale modelling.
2009 Elsevier B.V. All rights reserved.
Secondly, the δ7Li composition of the ocean (31.2‰, e.g. ) is significantly higher than any
Lithium has two stable and light isotopes (7.52% 6Li and 92.48% 7Li)
presently known Li source (the global riverine composition is
that fractionate significantly during silicate chemical erosion (weath-
estimated to possess a δ7Li value of 23.4‰ and that for hydrothermal
ering). The few studies of rivers undertaken thus far have suggested
exchange is 9‰, ). Two processes
that dissolved Li is mainly derived from silicates, even in large basins
have been proposed to account for Li isotope fractionation during
of mixed lithology, and that the Li isotope composition of waters
weathering: (i) rock or mineral leaching, (ii) the formation of
varies with indices of chemical erosion (
secondary minerals. Some soil studies suggest that 7Li is preferentially
released into solution from fine grained sediments
sedimentary records may therefore preserve unique information on
This is a priori surprising since 6Li
past silicate chemical erosion rates, particularly if the relationship
diffuses much quicker than 7Li and other studies suggest little isotope
between δ7Li in waters and silicate chemical erosion rate can be
fractionation during Li loss from a basalt (
quantified at a continental scale.
In contrast, both experiments and observa-
The main evidence for Li isotope fractionation accompanying
tions provide evidence for Li isotope fractionation during clay
silicate chemical erosion is firstly, that measured δ7Li ratios of
formation, at both low and high temperatures
continental waters (δ7Li = ((7Li/6Li) / (7Li/6Li)LSVEC − 1) ⁎ 1000) are
systematically higher than the rocks they drain and the sediments
they carry (suspended and bed load)
Small catchments or monolithological basins are characterized by
fewer variables, and provide a means to deconvolve the primarycontrols on silicate weathering rates. The volcanic island of Iceland isone of the few regions where river water chemistry and sediment
⁎ Corresponding author.
E-mail address: (N. Vigier).
fluxes have been extensively studied and monitored for more than
0012-821X/$ – see front matter 2009 Elsevier B.V. All rights reserved.
doi:
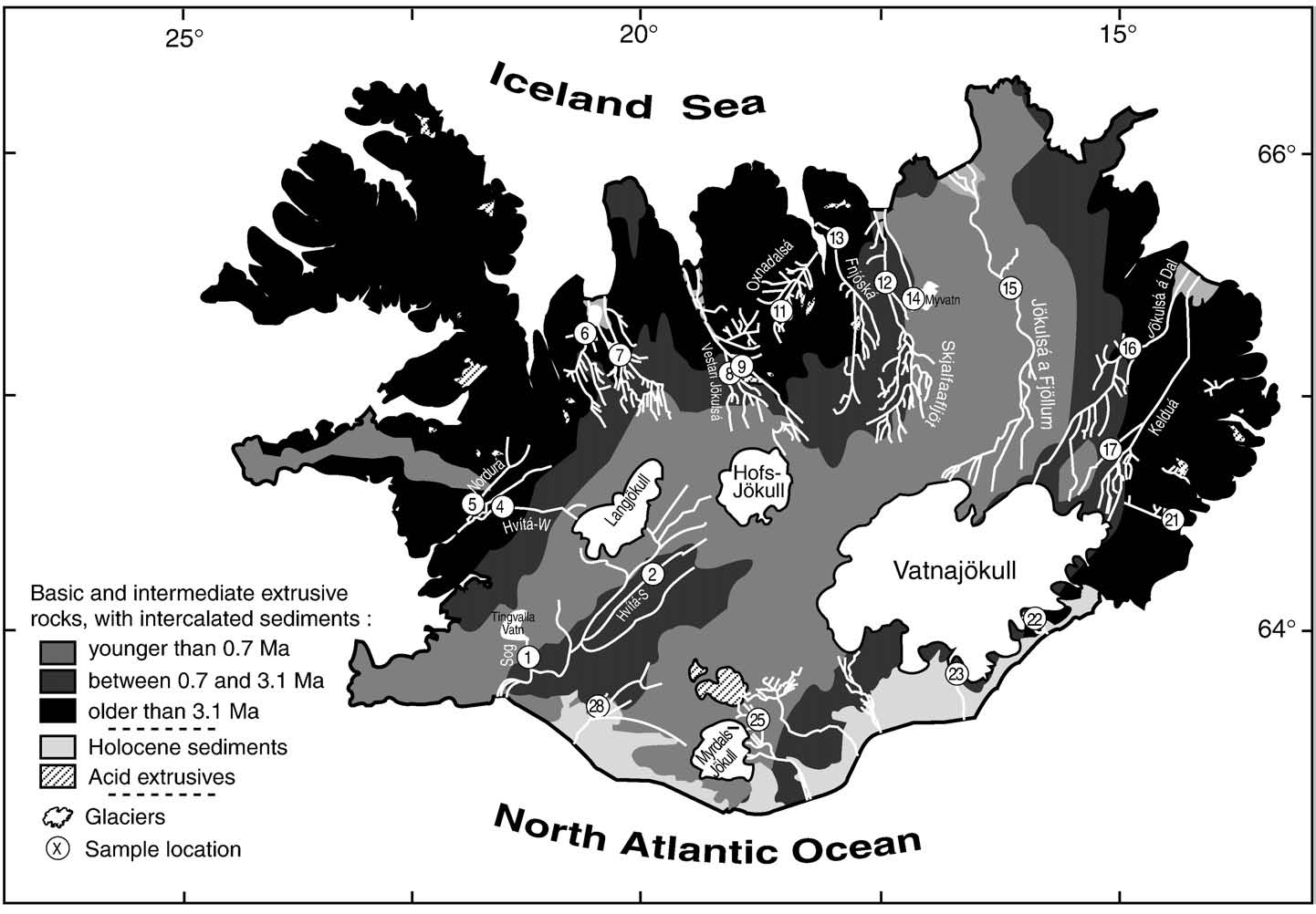
N. Vigier et al. / Earth and Planetary Science Letters 287 (2009) 434–441
30 years. Geology and rock composition are also well-constrained and
rivers have been recognized in Iceland: (i) spring-fed rivers, mainly
anthropogenic inputs are minimal. Weathering in Iceland has then
located in the central volcanic part where the high rock permeability
been extensively studied (e.g.
precludes significant surface runoff, (ii) glacier-fed rivers, and (iii) direct
runoff rivers draining mainly older basalts, where compaction and
). Recent studies have demonstrated a large
sealing by secondary minerals reduces the permeability. Soils are
range in chemical and physical erosion rates (
generally thin and the main secondary minerals in soils are allophane
Runoff and the age of the basalt
and ferrihydrite ().
appear to be two key controls on weathering. In addition, glass may
Quaternary volcanic rocks formed under ice during the last 3 Myr
play a major role because it can dissolve up to 6 times faster than
are glassy (hyaloclastites) but those formed when the island was ice-
crystalline basalt (e.g.
free are mostly in the form of crystalline lava flows. Thus, the most
and is abundant in the hyaloclastites of the central and
recent basalts are characterized by a glassy texture, in contrast with
glaciated part of the island.
the older northern lava flows which are mainly crystalline.
This study presents Li isotope data for waters and sediments of the
More than twenty rivers were sampled across Iceland in June/July
main Icelandic rivers. The samples are the same as those used for
2001 (). Catchments from the volcanically active zone in the
constraining chemical erosion rates in from
centre of Iceland, where the hydrothermal contribution to rivers can
dissolved major elements, allowing a direct comparison of chemical
be significant, were avoided. Glacier-fed rivers have also been
erosion and riverine δ7Li.
sampled, notably the Skaftafellsa River (#23) which is sourced directlyby the large Vatnajokull glacier. More details on the samples and their
locations can be found in and
River monitoring by the University of Iceland and the Hydrological
The island of Iceland is located on the Mid-Atlantic ridge. A
Division of the National Energy Authority provides precise estimates
combination of sea-floor spreading and associated volcanism has
of discharge and corresponding runoff for each watershed studied
produced a symmetrical SW–NE zonation in the age of the basalts,
here. In addition, the Total Suspended Sediment (TSS) load has been
from the centre of the island towards the east and north-west coasts.
regularly recorded for a number of Icelandic rivers over the last four
The older basalts (>3 Ma) are located in the north and east of the
island, while the most recent basalts are mainly located in the central
). Both records provide valuable information
and volcanically active zone.
that can be used to estimate the physical and chemical erosion rates of
The climate in Iceland is oceanic boreal, and less than 25% of the
most of the watersheds of the sampled rivers. For 2001, a wide range
island is vegetated and about 12% is covered by glaciers. Three types of
of physical erosion rates were inferred for Icelandic catchments,
Fig. 1. Map of the Icelandic rivers sampled during June 2001 for Li analyses. Sample numbers refer to sample designation as given in .
N. Vigier et al. / Earth and Planetary Science Letters 287 (2009) 434–441
between 21 and 4864 t/km2/yr, with an average of 519 t/km2/yr
sequence of analyses blanks are measured before and after each
which compares well with previous estimates This
sample and standard for background correction. Blank values are low,
is about twice the world average estimated by
typically 3–4 mV for the 7Li (i.e. 0.2%), and 5 min wash time is
. Chemical erosion rates, which can be estimated from the flux
sufficient to achieve a stable background value. Since blanks are very
of dissolved elements exported by rivers, are lower than physical
stable, the background correction applied to samples and standards is
erosion rates, ranging from 13 to 333 t/km2/yr. Chemical erosion
made using the average value of the two bracketing blanks.
rates correlate positively with physical erosion rates and runoff, and
The accuracy of this procedure has been checked using Li solutions
negatively with the mean age of the drained basalts (e.g.
made from LSVEC powder and 6Li and 7Li spikes of known
composition (Li6-N and Li7-N respectively, ),and reference materials. Two analyses of seawater gave δ7Li values of
3. Analytical procedure
31.25 ± 0.16 and 31.31 ± 0.12 respectively, which closely correspondto published values (e.g. see review in
The JB-2 basalt reference material has also been analyzedand gave δ7Li values of 5.65 ±0.12 and 5.73 ± 0.12, which are within
All river samples were filtered on the field using acetate cellulose
the published range for this basalt
filters (0.2 μm) which separated the dissolved phase from suspended
particles, collected on the filter with ultra-pure water. Li concentra-tions (along with other major and trace cations) were determined
using ICP-MS. For the suspended and bed load sediments, Li contentswere calibrated against a number of International rock reference
materials including AC-E (granite), WS-E (dolerite), and BIR-1, BCR-2and BHVO-1 (basalts). The external reproducibility, determined from
The Li contents of Icelandic river waters range between 20 ng l−1
repeat measurements of BCR-2, is better than 2% (1σ), where the
for the Heioarvatn and Geithellnadalur Rivers (#19 and #21), draining
measured concentration of Li in BCR-2 of 9.7 ± 0.2 ppm (1σ) is
old basalts in the eastern part of the island (basalt mean age 5.9 Ma),
indistinguishable from the certified value of 9 ± 2 ppm (USGS
and 2.2 μg l−1 for the Vestari Jökulsa River. This range is similar to that
Certificate of Analysis). For the dissolved load Li was calibrated
observed for rivers in a small watershed located in western Iceland
against an in-house standard (Scottish river water). The external
(). The mean Li content of Icelandic
reproducibility, determined by repeat measurement of the natural
rivers is 0.6 μg l−1. This is significantly lower than both the Li content
river water certified reference material SLRS-4, is better than 3%. The
of world-wide rivers (and the Li contents of rivers
measured Li content of SLRS-4 of 0.48 ± 0.01 ppb is similar to the value
draining mixed lithology basins of the Himalayas (
of 0.54 ± 0.07 ppb obtained by for the same
This is likely to be due to low Li contents in basalts relative to
river water.
granites and gneisses, and also to high runoffs in Iceland during the icemelting season. The Li contents of two samples from Geysir hot spring,
located in central Iceland, have also been determined and are 389 μg/l and 360 μg/l respectively.
Special caution was taken for ensuring complete dissolution of all
samples before the Li separation chemistry. River waters were
evaporated in Teflon beakers and the residues were dissolved severaltimes in concentrated nitric acid. Bulk sediments and suspended
Li isotope compositions have been determined for all river waters
particles were digested in a mixture of HF–HNO3–HCl and HClO4,
and some suspended sediment and bedload samples. The δ7Li values
following the procedure described in and
of the waters range between 10.1‰ for the Skaftafellsa (#23), a
previously used for measuring Th isotopes. Cation exchange resin
glacier-fed river in the south of Iceland, and 23.8‰ for the Vididalsa
was used to separate Li from the sample matrix, following a procedure
River (#6) located in northern Iceland. This range is similar to those
modified from that of The 100% recovery
published for world-wide and Himalayan rivers
was checked for each sample, considering analytical uncertainties. Li
The δ7Li of Geysir hot spring has also been
isotopes were measured on pure Li fractions, using the Open
measured and is 5.5‰. The range for sands and suspended sediments
University Nu-Instruments MC-ICP-MS for waters and the BRGM
is narrow (3.1‰–4.8‰) and close to the values estimated for
Neptune MC-ICP-MS for sediments. A Cetac Aridus desolvating
unweathered MORBs (e.g. but contrasts with the
nebuliser was used with the Nu Instruments while a quartz dual
values published by for other
spray chamber and a low flow PFA microconcentric nebuliser were
Iceland river sediments (−1.3‰ to 8.9‰).
used with the Neptune. More details concerning the analyticalprocedure are described in and in
. In brief, analyses were performed on 2–3% HNO3 solutionswith Li concentrations of 30 ng/ml, typically yielding a current
5.1. Sources of dissolved Li
intensity of 1.5 to 2 × 10−11 A for 7Li ion (using 1011 Ω resistors).
The total duration of data acquisition did not exceed 5–6 min,
In order to constrain the controls on the Li isotope signatures of
including sample uptake and peak centering. The analytical protocol
Icelandic river waters, it is important to first determine the sources of
employed involves the acquisition of 15 ratios with a 16 s integration
dissolved Li. The Li contents of Icelandic rivers are relatively low
time per ratio, and yields an in-run precision of 0.15‰ (2σm). The
(0.02–2.2 μg/l) and even though these rivers mainly drain basalts,
sample introduction rate is approximately 80–100 µl/min and the
alternative Li sources must be considered, in particular hydrothermal
total volume of sample used for each measurement is less than
waters and atmospheric input.
600 µl, corresponding to about 15–20 ng of Li. Sample are bracketed
The rivers studied here are mainly located away from the central
by the L-SVEC RM 8545 standard in order to correct isotopic
and active ridge where most of the hydrothermal activity is known to
compositions for instrumental mass fractionation. The measured 7Li/
occur. Nevertheless, Li concentrations are several orders of magnitude
6Li ratio of a sample is normalized to the mean 7Li/6Li ratio of the two
greater in hydrothermal waters than in river waters. In the ocean, Li
standards run immediately before and after. In addition, during each
contents of hydrothermal fluids range from 3000 to 9000 μg/l. In
N. Vigier et al. / Earth and Planetary Science Letters 287 (2009) 434–441
Iceland, the Li content of geothermal waters ranges from 35.9 μg/
sources for these elements. In mixing diagrams, river waters all plot
l to 6600 μg/l (
between the three endmembers considered: precipitation (or glacier-
). In the thermal waters of Geysir, which represents the most
fed waters), hydrothermal springs and basalt weathering ).
famous, and one of the largest, hydrothermal fields of Iceland, two
However, as discussed above, atmospheric inputs are likely to be
aliquots sampled during different seasons yield Li contents of 389 μg/
small when compared to the amount of Li released by basaltic
l and 360 μg/l. In contrast with Li concentrations, the δ7Li measured in
lithologies during weathering. The waters possessing high δ7Li and K/
hydrothermal waters of mid-ocean ridge systems are relatively
Li values are located in the older and drier parts of the island. These
homogeneous, around 8.7‰ ± 1.4‰ (The δ7Li
areas are characterized by a significant amount of clays and secondary
measured at Geysir spring is 5.5‰. Taking these values into account, it
minerals that have replaced primary minerals in the weathered lava
can be calculated that a hydrothermal source contribution of less than
flows. Li uptake by secondary minerals could also be responsible for
1% would impose similar δ7Li values for all the rivers studied here.
elevated K/Li, Na/Li and Mg/Li ratios and it is therefore not possible,
Thus, the wide range of δ7Li values displayed by Iceland river waters a
based on concentrations only, to distinguish between this effect and a
priori suggests that the contribution from hydrothermal inputs must
mixing process involving the endmembers described above ().
be significantly less than 1%.
Moreover, as illustrated in a and b, it is also difficult, based on
It has previously been shown that melted ice from central glaciers
concentrations alone, to distinguish between the role of basalt
can be used as a proxy for atmospheric inputs in Iceland (e.g.
dissolution and the potential impact of any hydrothermal springs
). The Li content of melted ice is relatively low (<6 ng/l),
drained by these rivers.
consistent with the non-volatile nature of Li during low temperature
Since the range in δ7Li values in river waters (10.1‰–23.8‰) lies
evaporation processes. However, this is not negligible when com-
between those of hydrothermal ( 9‰) and atmospheric inputs
pared with the Li contents measured in some of the Icelandic rivers,
(31.2‰), it might be argued that a mixing process involving these
such as the Heioarvatn and Geithelnadalur Rivers (). It can be
two sources could explain the entire range of isotope compositions
reasonably assumed that most of the atmospheric Li comes from
measured for these waters. In such a case, it would be very difficult to
marine aerosols and that the δ7Li composition of any precipitation is
infer precise information concerning low temperature basalt weath-
close to the seawater value (31.2‰,
ering rates from the Li isotope signatures of Icelandic rivers. However,
In river waters, the highest δ7Li (>21‰) and K/Li values,
illustrates that the δ7Li values in river waters cannot be
closest to the composition of precipitation (), are located in the
explained by simple binary mixing of these sources. Similarly, mixing
area with the lowest runoff and where the percentages of glacial cover
between atmospheric inputs and a solution resulting from basalt
are the lowest at the basin scale (Consequently, the
weathering does not explain the river δ7Li River δ7Li values
high δ7Li values and K/Li ratios measured in these rivers cannot
are systematically higher than any of the theoretical mixing lines. The
simply be explained by a greater contribution from precipitation.
highest δ7Li values correspond to the oldest basins with the lowest
The sources of Li in Icelandic rivers can be more precisely
runoff and are therefore unlikely to result from a greater contribution
constrained by comparing the concentrations of Li and major
from the atmosphere. High δ7Li values, associated with high K/Li or
elements in river waters with three determined endmembers (basalt
Na/Li ratios, are more consistent with a Li uptake process, during the
weathering, precipitation, hydrothermal exchange) (a,b,c). To a
formation of secondary phases. shows that the initial solution
first approximation, basalt weathering in Iceland can be assumed to
must have low δ7Li and a high Li content. It might be envisioned that
be congruent, as has been demonstrated for some of the youngest
this process occurs in soils and that initial soil solutions would either
Icelandic basins (e.g. leading to waters with K/Li,
have isotopic compositions close to that of the source basalt, with or
Mg/Li and Na/Li ratios similar to the average drained basalt
without similar K/Li ratios, or would lie on the mixing curve with
). The Li contents of the rivers correlate with K, Na and Mg
precipitation. These data also indicate that negligible isotope
contents (r2 = 0.60–0.75, not shown here), suggesting identical
fractionation occurs during basalt leaching, as has been suggested in
Table 1Li isotope composition (δ7Li) of Icelandic river waters (w), suspended sediments (p) and bedload sands (s).
(t km−2 yr−1)
Vestari Jokulsa, Goddolum
Laxa at Myvatssveit
Jökulsa a Fjöllum
Jökulsa i Fljotsdal
Age and chemical erosion rates are from
a Mg, K and Na concentrations for waters are from b Sample taken at the output of the Myvatn lake.
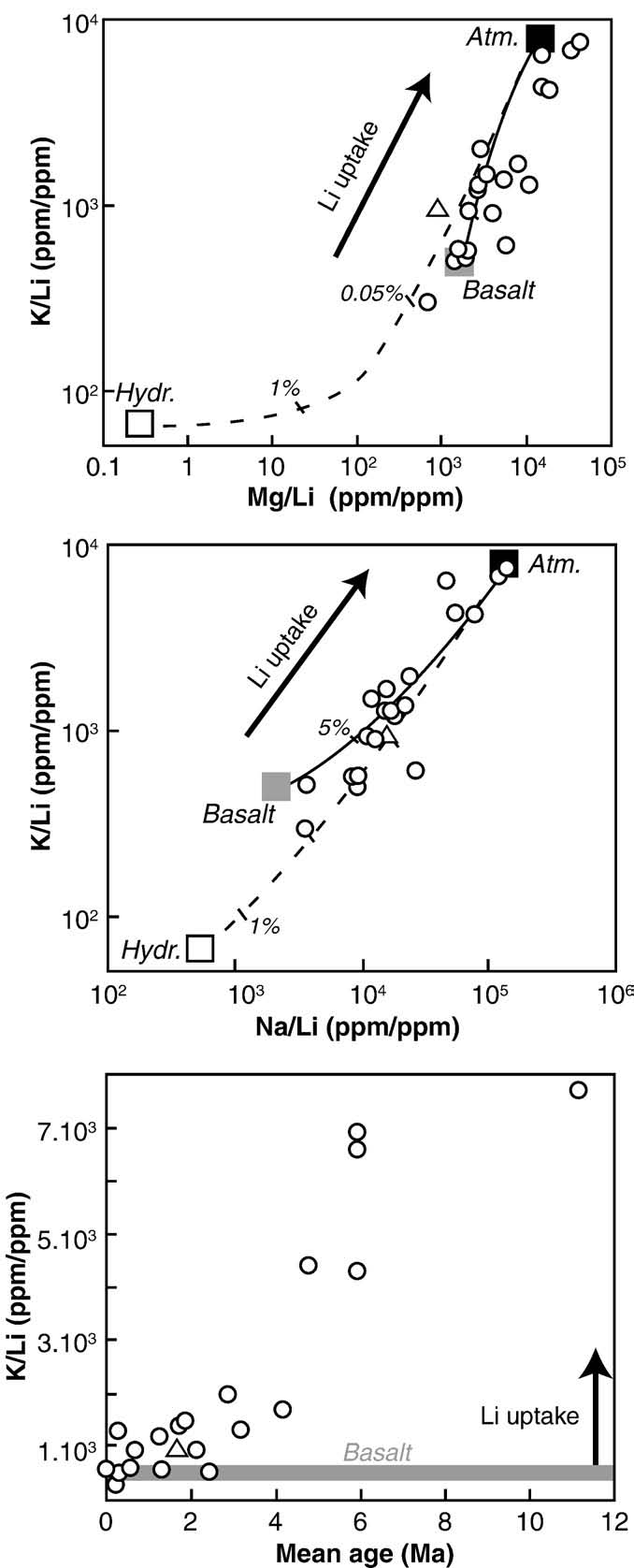
N. Vigier et al. / Earth and Planetary Science Letters 287 (2009) 434–441
a previous study Indeed a leaching
values. However, the main positive trend resulting from secondary
process associated with preferential release of 7Li in waters (relative
mineral formation does not appear to have been significantly disturbed
to 6Li) would result in a negative trend on .
by hydrothermal processes, as expected given the sampling strategy
A hydrothermal contribution to the rivers cannot be completely
adopted in this study (see ).
ruled out, particularly for glacier fed rivers (Skaftafellsa, #23), whilehydrothermal springs may also be located along the course of other
5.2. Assessing the link between riverine δ7Li and silicate erosion rates
rivers. Such a contribution, if significant, would decrease river δ7Li
As illustrated in it is difficult to account for the δ7Li values
measured in river waters by mixing processes alone. In the older partsof Iceland, high δ7Li values in rivers are associated with low Licontents. These older terrains are characterized by low chemicalerosion rates and basalt porosity has been significantly reduced by theprecipitation of secondary minerals (e.g. Incontrast, in the younger areas, chemical erosion rates are greater,corresponding to a more congruent weathering process, due inparticular to the higher susceptibility to weathering of glassyhyaloclastites. Taken together, these observations strongly suggest alink between the nature and the intensity of silicate chemical erosionand Li isotope signature in river waters. In fact, excepted the watersampled at the output of the Myvatn lake, a negative correlation canbe observed between δ7Li and chemical erosion rates estimated basedon major elements measured for the same samples , This is the first direct correlation between both parameters andconfirm the initial suggestion of , that the δ7Licomposition of river waters could yield precious information onchemical erosion rates, both past and present. An empirical lawlinking δ7Li and silicate chemical erosion rate (w) can be inferred fromthe data shown in :
δ7Li = −4:8ðF1:8Þ · lnðwÞ + 36ðF5:9Þ
If this law fits well the data for silicate chemical erosion rates lower
than 100 t km− 2 yr− 1, i.e. for most of the basins world-wide(), it would need to be refined for higherweathering rate areas. In order to use this relationship for global scalebio-geochemical modelling, more data are needed in order to confirmif this law is representative of silicate chemical weathering at a largerscale, and in particular for other rock types and other climate regimes.
Indeed, if secondary mineral formation is the key process, reducing
the levels of cation fluxes to the ocean and resulting in high river δ7Livalues, then quantitative application of this system necessitates thedetermination of Li isotope fractionation accompanying the formationof different Li rich secondary minerals formed during continentalweathering. However, as suggested by , Li isotopefractionation is expected to be broadly similar for all tri- and di-octahedral clays, and solution chemical composition has no effect onisotope fractionation during smectite formation. If this is confirmed,then the law described by Eq. (1) may be representative at a muchlarger scale than simply that of Iceland. It is instructive to note that, bycombining silicate weathering rates reported by and δ7Li values reported by , most of the largerivers, draining lithologies typical of continental crust, display highδ7Li and correspond to very low chemical erosion rates. The Amazon,Ganges, Orinoco, Lena, Huanghe, and the Qiantang Rivers all display(at their outlet) δ7Li values greater than 21‰ for corresponding
Fig. 2. Dissolved K/Li versus Mg/Li, Na/Li (ppm/ppm) and the mean age of the drainedbasalts for the main Icelandic rivers. The mean compositions of atmospheric input(Atm.), hydrothermal springs (Hydr.) and drained basalts (Basalt) are also reported(data from analyses of melted Icelandic glacier ice, Pogge von Strandmann (pers.
comm.), and respectively). Dashed lines represent theoretical mixingcurves between hydrothermal springs and atmospheric inputs, and plain linesrepresent theoretical mixing curves between a water resulting from the congruentweathering of basalts and atmospheric inputs. Arrows represent Li uptake by secondaryminerals from a water resulting from the congruent weathering of basalts. The slope ofthese arrows and the slope defined by the data are close to unity, implying that when Liis taken up by clays, K, Na and Mg are similarly taken up. The triangle is for the glacierfed river Skaftafellsa (#23).
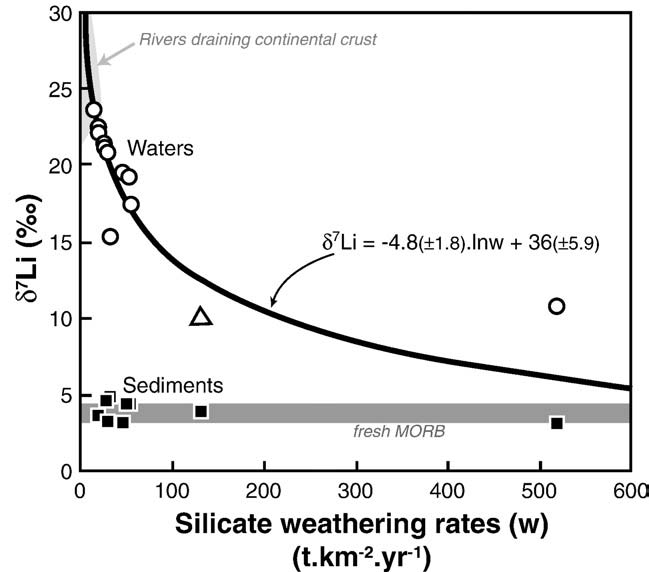
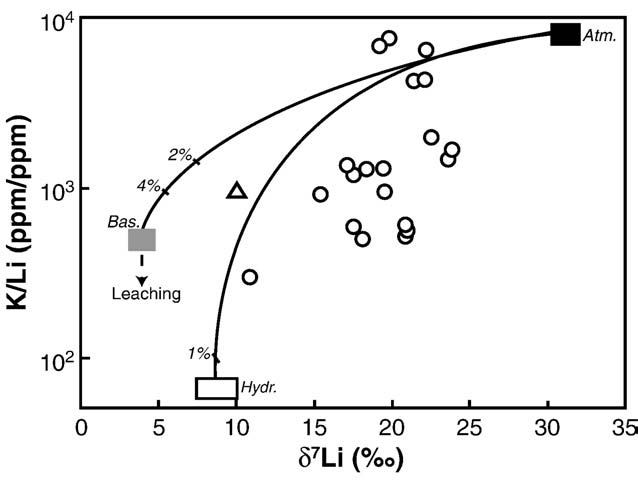
N. Vigier et al. / Earth and Planetary Science Letters 287 (2009) 434–441
described by Eq. (4), is calculated assuming a steady-state processsuch that the erosion products carried by the river reflect the sourcerock composition (e.g.
δ7Lirock = fs · δs + fw · δw
where δ7Lirock is constant and equivalent to the value of unweatheredMORB. This steady-state has recently been demonstrated in Icelandfrom U-series isotopes measured in rivers ), andfrom major and trace element data ().
As shown in , this simple model of Li uptake by secondary
minerals can explain all the river data (waters and sediments) whenδ7Lisediment–water ranges between −1‰ and −7.5‰, and fw is less than 20%. The precise mechanisms responsible for the isotopic composi-tion of river waters and sediments are likely to be more complex thanthose proposed here, but this model presents an alternative to theleaching model proposed for explaining soil profiles and water data
Fig. 3. K/Li versus
(e.g. The degree of Li
δ7Li measured in the dissolved phases of the main Icelandic rivers,
with same end-members as in The black arrow represents the evolution of water
isotope fractionation required to explain the data is significantly
composition in the case of leaching from a basaltic rock, assuming no associated Li
lower than the estimation of (−17‰) for a clay
isotope fractionation. Mixing lines do not fit the river data: measured δ7Li are
mixture composed of smectite, zeolite, palagonite and oxyhydroxides
systematically larger than predicted by mixing processes.
formed at 4 °C on the ocean seafloor. However, isotope fractionationassociated with weathering in Iceland may be influenced by other
chemical erosion rates lower than 14 t km−2 yr−1. Thus, most of the
types of secondary minerals. Moreover adsorption processes and
large rivers draining continental silicates plot on broadly the same
coefficients are likely to be different in seawater and continental
trend to that defined by the Icelandic rivers (see ). This may
environments. Recent experimental study shows that the incorporation
imply that Li isotopes do not fractionate during the leaching of Li rich
of Li into the octahedral sites of a smectite at temperatures lower than
mineral phases present on continents, in particular biotites. However,
90 °C is associated with Li isotope fractionations ranging between 8.8‰
this would need to be checked experimentally. In order to derive a
and 10.9‰ In parallel, Li adsorption on smectite may
more general law, it would be necessary to measure δ7Li on the same
be associated with lower or negligible isotope fractionation (
water samples as those used for estimating chemical erosion rates and
). Further experiments are
to perform systematic studies of granitic catchments.
needed to resolve the present lack of knowledge concerning theprocesses and phases that fractionate Li isotopes at low temperatures.
5.3. Modelling clay–water Li isotope fractionation
Overall, the Li isotope composition of river waters appears to be
related to chemical erosion rates and secondary mineral phase
On a regional scale, the δ7Li values of the dissolved phase in
formation. Several studies have shown that the stability of secondary
Icelandic rivers are highly variable. In contrast, the corresponding
phases in Iceland is dependent upon a number of interrelated factors,
suspended and bedload sediments display much more homogeneous
the principal of which are elemental supply via leaching, water
δ7Li values, close to the composition of unweathered MORB. Wheredata for both phases are available, such a relationship, betweendissolved and sediment loads has been observed for most rivers todate, in particular for Himalayan catchments and the Orinoco basinA simple Rayleighfractionation model can explain the relationship between thesephases, taking into account both the steady-state nature of theerosion processes in Iceland and clay formation. Li is first leached fromthe basaltic source rocks (e.g. in soils) without significant isotopefractionation, resulting in a dissolved phase with the same δ7Li as theunweathered parent rock (i.e. δ7Li ≈ 4‰). When secondary mineralsare formed, they incorporate a significant fraction of the dissolved Li.
These secondary minerals possess a light δ7Li isotope composition,thus their formation will both deplete the residual waters in 6Li, anddrive their δ7Li values to heavier compositions. It is possible to verify ifsuch a single process could produce both water and sediment riverdata, using the following the equations:
δw = δiw + 1000:ðα−1Þ · ln fw
δs = δw + 1000 · ln α
s = ½ðδiw 1000 + 1Þ · ð f α
1Þ = ð fw 1Þ−1 · 1000
Fig. 4. Chemical erosion rates estimated from major elements in Icelandic river watersversus δ7Li measured in the same waters (open symbols) and in the
corresponding river sediments (black symbols). The lake sample (#14) is not
w and δs are the δ7Li of the dissolved phase and sediment
represented. The curve and equation represent the best fit for the Icelandic water
respectively, fw is the fraction of residual dissolved Li, and α is the
data. The published values for river waters draining large areas of continental crust are
clay–water Li isotopic fractionation factor (δ7Liclay–water =1000ln(α)).
shown for comparison (shaded field) (Amazon, Orenoque, Ganges, Lena, Huanghe,
The composition of the cumulated or "bulk" sediment (δ–s), as
Qiantang; ) (see text for more details).
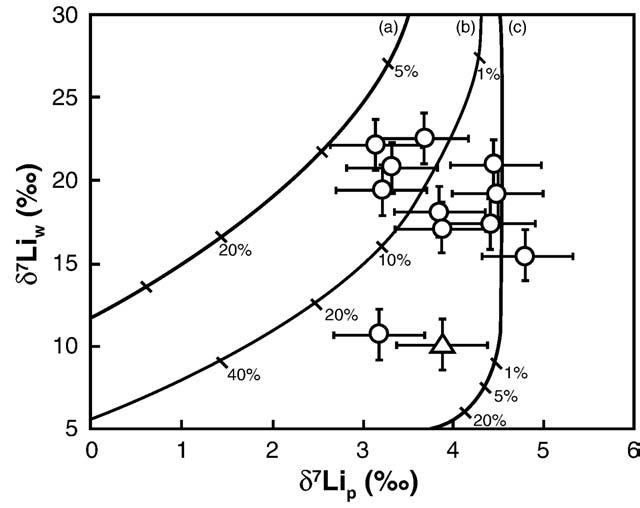
N. Vigier et al. / Earth and Planetary Science Letters 287 (2009) 434–441
global scale and may be of particular use for estimating past changesin continental weathering. Further data are now needed in order todetermine if this law can be applied to different rock types anddifferent climatic regimes.
We would particularly like to thank Christian France-Lanord
(CRPG) for fruitful discussion, and Peter Van Calsteren, Louise Thomasand Mabsie Gilmour (OU) for their technical help during wateranalyses. This project has been funded by the Open University and bythe "Reliefs de la Terrre" INSU-CNRS program.
Arnalds, O., 2004. Volcanic soils of Iceland. CATENA 56 (1–3), 3–20.
Arnorsson, S., Gunnarsson, I., Stefansson, A., Andresdottir, A., Sveibjornsdottir, A., 2002.
Major element chemistry of surface- and ground waters in basaltic terrain, N-Iceland. I. Primary mineral saturation. Geochim. Cosmochim. Acta 66 (23),
Fig. 5. δ7Li of the dissolved (δ7Liw) and sediment (δ7Lip) loads of the main Icelandic
rivers. The curves represent Rayleigh evolution in the case of Li uptake from an initial
Carignan, J., Cardinal, D., Eisenhauer, A., Galy, A., Rehkämper, M., Wombacher, F., Vigier,
solution with the same isotopic composition of the source basalts (see Eqs. (2)–(5) in
N., 2004. A reflection on Mg, Cd, Ca, Li and Si isotopic measurements and related
text), and clay–water Li isotope fractionation of (a) −7.5‰, (b) −5‰ and (c) −1‰.
reference materials. Geostand. Geoanal. Res. 28, 139–148.
Carignan, J., Vigier, N., Millot, R., 2007. Three secondary reference materials for Li
isotope measurements: Li7-N, Li6-N and LiCl-N solutions. Geostand. Geoanal. Res.
temperature, pH and residence time. For most of the samples studied
here, taken from the lower reaches of their respective catchments, a
Chan, L.-H., Edmond, J.M., 1988. Variation of lithium isotope composition in the marine
steady state is likely to have been achieved such that soil residence
environment: a preliminary report. Geochim. Cosmochim. Acta 52, 1711–1717.
Chan, L.-H., Edmond, J.M., Thompson, G., Gillis, K., 1992. Lithium isotopic composition of
times are constant and clay formation is compensated for by chemical
submarine basalts: implications for the lithium cycle to the ocean. Earth Planet. Sci.
and physical erosion. However, a steady state may not always be
Lett. 108, 151–160.
attained, for example in glacier-fed rivers (such as the Skaftafellsa
Chan, L.-H., Edmond, J.M., Thompson, 1993. A lithium isotope study of hot springs and
metabasalts from mid ocean ridge hydrothermal systems. J. Geophys. Res. 98,
River (#23)) or in groundwaters, where high pH results as much from
the isolation from atmospheric CO2, as from chemical weathering. In
Chan, L.-H., Gieskes, J.M., You, C.F., Edmond, J.M., 1994. Lithium isotope geochemistry of
such cases, these may be no simple relationship with chemical
sediments and hydrothermal fluids of the Gaymas Basin, Gulf of California.
Geochim. Cosmochim. Acta 58, 4443–4454.
weathering rates, even if secondary minerals such as Fe–Mg–Ca
Chan, L.-H., Alt, J.C., Teagle, D.A.H., 2002. Lithium and lithium isotope profile through
smectites and zeolites are stable.
the upper oceanic crust: a study of seawater–basalt exchange at ODP Sites 504B
These observations indicate that the model presented in this study
and 896A. Earth Planet. Sci. Lett. 201, 197–201.
Elliott, T., Thomas, A., Jeffcoate, A., Niu, A., 2006. Lithium isotope evidence for
enables the link between secondary mineral formation, chemical
subduction enriched mantle in the source of mid-ocean-ridge basalts. Nature 443,
erosion rates and the Li isotope composition of residual waters to be
quantified, and the data also suggest that secondary mineral
Eiriksdottir, E.S., Louvat, P., Gislason, S.R., Óskarsson, N., Hardardóttir, J., 2008. Temporal
formation acts as a key control on the cation flux to the ocean. The
variation of chemical and mechanical weathering in NE Iceland: evaluation of asteady-state model of erosion. Earth Planet. Sci. Lett. 272, 78–88.
relationship between water chemistry and secondary mineral
Gaillardet, J., Dupré, B., Louvat, P., Allègre, C.J., 1999. Global silicate weathering and CO2
formation is consistent with the U-series data from the same rivers,
consumption rates deduced from the chemistry of large rivers. Chem. Geol. 159,
where soil development and sediment residence time have also been
Gannoun, A., Burton, K.W., Vigier, N., Gislason, S.R., Rogers, N., Mokadem, F., Sigfusson,
shown to be a major influence on chemical erosion and river water
B., 2006. The influence of weathering process on riverin osmium isotopes in a
basaltic terrain. Earth Planet. Sci. Lett. 243, 732–748.
Georg, R.B., Reynolds, B.C., West, A.J., Burton, K.W., Halliday, A.N., 2007. Silicon isotope
variations accompanying basalt weathering in Iceland. Earth Planet. Sci. Lett. 261,
6. Summary and conclusion
Gislason, S.R., Eugster, H.P., 1987a. Meteoric water–basalt interactions. II: a field study
The Li isotope compositions of the dissolved phase and suspended
in N.E. Iceland. Geochim. Cosmochim. Acta 51 (10), 2841–2855.
Gislason, S.R., Eugster, H.P., 1987b. Meteoric water–basalt interactions. I: a laboratory
and bedload sediments of the main Icelandic rivers have been
study. Geochim. Cosmochim. Acta 51 (10), 2827–2840.
determined by MC-ICP-MS. For the dissolved phase, δ7Li range
Gislason, S.R., Arnorsson, S., Armannsson, H., 1996. Chemical weathering of basalt in
between 10.1‰ and 23.8‰, while river sediments display a narrower
southwest Iceland: effects of runoff, age of rocks and vegetative/glacial cover. Am. J.
Sci. 296, 837–907.
range of values (3.1‰–4.8‰), close to that of unweathered MORB.
Gislason, S.R., Oelkers, E.H., Snorrason, A., 2006. Role of river-suspended material in the
High δ7Li values are associated with high K/Li, Na/Li and Mg/Li ratios,
global carbon cycle. Geology 34 (1), 49–52.
and characterise the waters draining mainly old and weathered
Henley, R.W., Ellis, A.J., 1983. Geothermal systems ancient and modern: a geochemical
review. Earth Sci. Rev. 19, 1–50.
basalts. Rivers with low δ7Li are located in the younger parts of the
Huh, Y., Chan, L.-H., Zhang, L., Edmond, J.M., 1998. Lithium and its isotopes in major
island. It is shown that source-mixing alone, between precipitation,
world rivers: implications for weathering and the oceanic budget. Geochim.
Li-rich hydrothermal springs and basalt weathering, cannot explain
Cosmochim. Acta 62, 2039–2051.
the entire range of δ7Li values. Instead, the Li isotope compositions of
Huh, Y., Chan, L.-H., Edmond, J.M., 2001. Lithium isotopes as a probe of weathering
processes: Orinoco River. Earth Planet. Sci. Lett. 194, 189–199.
both waters and sediments can be explained by a steady-state erosion
Huh, Y., Chan, L.-H., Chadwick, O., 2004. Behavior of lithium and its isotopes during
process where the formation of secondary minerals is associated with
weathering of Hawaiian basalts. Geochem. Geophys. Geosys. 5. doi:10.1029/
significant Li isotope fractionation. The data obtained in this study,
James, R.H., Palmer, M., 2000. Marine geochemical cycles of the alkali elements and
combined with chemical erosion rates previously determined on the
boron: the role of sediments. Geochim. Cosmochim. Acta 64, 3111–3122.
same samples provide the first direct evidence for a relationship
James, R.H., Rudnicki, M.D., Plamer, M., 1999. The alkali element and boron
between δ7Li and chemical erosion rates. An empirical law between
geochemistry of the Escanaba Trough sediment-hosted hydrothermal system.
Earth Planet. Sci. Lett. 171, 157–169.
chemical erosion rate and δ7Li can be defined, and comparison with
James, R.H., Allen, D.E., Seyfried, Jr, 2003. An experimental study of oceanic crust and
literature data suggests that this law may be applicable at a more
terrigenous sediments at moderate temperatures (51 to 350 °C): insights as to
N. Vigier et al. / Earth and Planetary Science Letters 287 (2009) 434–441
chemical processes in near-shore ridge-flank hydrothermal systems. Geochim.
Stefansson, A., Gislason, G.R., 2001. Chemical weathering of basalts, SW Iceland: effect
Cosmochim. Acta 67, 681–691.
of rock crystallinity and secondary minerals on chemical fluxes to the ocean. Am. J.
Jeffcoate, A., Elliott, T., Thomas, A., Bouman, C., 2004. Precise, small sample size
Sci. 6 (513–556), 2001.
determinations of lithium compositions of geological reference materials and
Taylor, S.R., Urey, H.C., 1938. Fractionation of the lithium and potassium isotopes by
modern seawater by MC-ICPMS. Geostand. Geoanal. Res. 28, 161–172.
chemical exchange with zeolites. J. Chem. Phys. 6, 429–438.
Kisakürek, B., Widdowson, M., James, R.H., 2004. Behaviour of Li isotopes during
Tomasson, H., 1986. Glacial and volcanic shore interactions. Part 1: on land. In:
continental weathering: the Bidar laterite profile, India. Chem. Geol. 212, 27–44.
Sigbjarnarson, G. (Ed.), Iceland Coastal and River Symposium Proceedings.
Kisakürek, B., James, R.H., Harris, N.B.W., 2005. Li and δ7Li in Himalayan rivers: proxies
University of Iceland, Reykjavik, pp. 7–16.
for silicate weathering? Earth Planet. Sci. Lett. 237, 387–401.
Vigier, N., Bourdon, B., Turner, S., Allègre, C.J., 2001. Erosion timescales derived from U-
Louvat, P., 1997. Etude géochimique de l'érosion fluviale d'îles volcaniques à l'aide des
decay series measurements in rivers. EPSL 193, 549–563.
bilans d'éléments majeurs et traces, Doctorat Thesis, IPGParis.
Vigier, N., Burton, K.W., Gislason, S.R., Rogers, N.W., Duchene, S., Thomas, L., Hodge, E.,
Millot, R., Guerrot, C., Vigier, N., 2004. Accurate and high-precision measurement of
Schaefer, B., 2006. The relationship between riverine U-series disequilibria and
lithium isotopes in two reference materials by MC-ICP-MS. Geostand. Geoanal. Res.
erosion rates in a basaltic terrain. Earth Planet. Sci. Lett. 249, 258–273.
28, 153–159.
Vigier, N., Decarreau, A., Millot, R., Carignan, J., Petit, S., France-Lanord, C., 2008.
Milliman, J.D., Meade, R.H., 1983. World-wide delivery of river sediment to the oceans.
Quantifying Li isotope fractionation during smectite formation and implications for
J. Geol. 91, 1–21.
the Li cycle. Geochim. Cosmochim. Acta 72, 780–792.
Négrel, P., Allègre, C.J., Dupré, B., Lewin, E., 1993. Erosion sources determined by
Williams, L.B., Hervig, R.L., 2005. Lithium and boron isotopes in illite–smectite: the
inversion of major and trace element ratios and strontium isotopic ratios in river:
importance of crystal size. Geochim. Cosmochim. Acta 69, 5705–5716.
the Congo Basin case. Earth Planet. Sci. Lett. 12, 59–76.
Wolff-Boenisch, D., Gislason, S.R., Oelkers, E.H., 2006. The effect of crystallinity on
Palsson, S., Vigfusson, G.H., 1991. Nidurstödur svifaurswmaelinga 1963–1990,
dissolution rates and CO2 consumption capacity of silicates. Geochim. Cosmochim.
Acta 70, 858–870.
Pistiner, J.S., Henderson, G., 2003. Lithium isotope fractionation during continental
Yeghicheyan, D., Carignan, J., Valladon, M., Bouhnik Le Coz, M., Le Cornec, F., Castrec-
weathering processes. Earth Planet. Sci. Lett. 214, 327–339.
Rouelle, M., Robert, M., Aquilina, L., Aubry, E., Churlaud, C., Dia, A., Deberdt, S.,
Pogge von Strandmann, P.A.E., Burton, K.W., James, R.H., van Calsteren, P., Gislason, S.R.,
Dupré, B., Freydier, R., Gruau, G., Hénin, O., de Kersabiec, A.-M., Macé, J., Marin, L.,
Mokadem, F., 2006. Riverine behaviour of uranium and lithium isotopes in an
Morin, N., Petitjean, P., Serrat, E., 2001. A compilation of silicon and thirty one trace
actively glaciated basaltic terrain. Earth Planet. Sci. Lett. 251, 134–147.
elements measured in the natural river water reference material SLRS-4 (NRC–
Rudnik, R.L., Tomascak, P.B., Njo, H.B., Gardner, L.R., 2004. Extreme lithium isotopic
CNRC). Geostand. Newsl. Geost. Geoanal. Res. 25, 465–474.
fractionation during continental weathering revealed in saprolites from South
Zhang, L., Chan, L.H., Gieskes, J.M., 1998. Lithium isotope geochemistry of pore waters
Carolina. Chem. Geol. 212, 45–57.
from Ocean Drilling Program Sites 918 and 919, Irminger Basin. Geochim.
Seyfried Jr., W.E., Chen, X., Chan, L.-H., 1998. Trace element mobility and lithium isotope
Cosmochim. Acta 62, 2437–2450.
exchange during hydrothermal alteration of seafloor weathered basalt: anexperimental study at 350 °C, 500 bars. Geochim. Cosmochim. Acta 62, 949–960.
Source: http://www.crpg.cnrs-nancy.fr/spip.php?action=acceder_document&arg=223&cle=6817cf05d7afe4dc41475845d4009033a0caa575&file=pdf%2F2025.pdf
ACEMBL Expression System User Manual Vers. 09.11 Yan Nie, Christoph Bieniossek, Imre Berger ACEMBL was developed at the European Molecular Biology Laboratory EMBL Grenoble Outstation 38042 Grenoble CEDEX 9, France Grenoble, August 21, 2009 ACEMBL System User Manual EMBL Grenoble, 2009 Table of Contents
instructieboek / Instruction leaflet / Bedienungsanleitung / livret d'instructions/ Manuale di istruzioni / folleto de instrucciones / Manual de instruções PAC 12&14 MOBILE Air conditioner/Dehumidifier MOBILE Klimaanlage/Luftentfeuchter Condizionatore/umidificatore MOBILE Aire acondicionado / deshumidificador PORTÁTIL