Pii: s0305-1978(02)00029-7
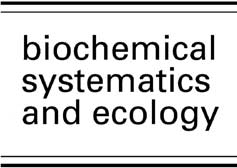
Biochemical Systematics and Ecology 30 (2002) 837–854
Phytochemical variation within populations of
Echinacea angustifolia (Asteraceae)
Shannon E. Binns a, John T. Arnason a,∗, Bernard R. Baum b
a Biology Department, University of Ottawa, 30 Marie Curie St., Ottawa, ON K1N 6N5, Canada
b Eastern Cereal and Oilseed Research Centre, Agriculture and AgriFood Canada,
Ottawa ON K1A 0C6, Canada
Received 23 November 2001; accepted 14 December 2001
Quantitative evaluation of phytochemical diversity in Echinacea angustifolia DC. popu-
lations from different natural geographic areas supports the existence of distinct natural chemo-types within the species. Consumers, growers and manufacturers of phytomedicines are inter-ested in chemotype identification for prediction of phytochemical content in cultivardevelopment. Six month old E. angustifolia roots, grown from nine different wild seed sourcesin a controlled environment, were extracted into 70% ethanol and 28 reported phytochemicalswere measured by HPLC separation. Two-way ANOVA between the nine populations revealedquantitative differences (p⬍0.05) in the caffeic acid derivatives 2,3-O-dicaffeoyl tartaric acid(cichoric acid), 2-O-caffeoyl tartaric acid (caftaric acid), 1,3-dicaffeoyl-quinic acid (cynarin),echinacoside and ten reported alkamides. Canonical discriminant analysis determined the phy-tochemical variables which contributed the most towards chemotype distinction for five of thenine populations: undeca-2E,4Z-diene-8,10-diynoic acid-2-methylbutylamide∗, dodeca-2E,4E-dienoic acid isobutylamide∗, dodeca-2E-ene-8,10-diynoic acid isobutylamide∗∗, hexadeca-2E,9Z-diene-12,14-diynoic acid isobutylamide∗, cichoric acid∗∗, caftaric acid∗, and echinaco-side∗∗ (∗p⬍0.0001, ∗∗p⬍0.05). Five of those compounds were also significantly associatedwith latitudinal variation by regression analyses (p⬍0.05). 2002 Published by ElsevierScience Ltd.
Keywords: Echinacea angustifolia; Secondary metabolism; Phytochemical variation; Chemoraces; Alkam-ides; Caffeic acid derivatives; Polyacetylenes
∗ Corresponding author. Fax: +1-613-562-5765.
E-mail address: [email protected] (J.T. Arnason).
0305-1978/02/$ - see front matter 2002 Published by Elsevier Science Ltd.
PII: S 0 3 0 5 - 1 9 7 8 ( 0 2 ) 0 0 0 2 9 - 7
S.E. Binns et al. / Biochemical Systematics and Ecology 30 (2002) 837–854
Plants of the genus Echinacea are native to North America, with wild populations
ranging from the US Great Plains north to the Canadian prairies, east to theAppalachian uplands and the southeastern coastal plains (McGregor, 1968). As oneof the most geographically-widespread species in the genus, E. angustifolia hasadapted naturally to different habitats from Texas to Saskatchewan, but it remainsone of the more difficult Echinacea species to cultivate successfully. Traditionally,the roots of E. angustifolia were the most frequently-used medicine among mostFirst Nations groups of the Great Plains region (Kindscher, 1989; Shemluck, 1982).
In the last decade, Echinacea species have regained this popularity as the top-sellingmedicinal market botanical (Brevoort, 1998). Wild-harvested E. angustifolia rootshave the highest market value of all Echinacea material sold as phytomedicine.
Since worldwide economic demand for E. angustifolia in particular has far
exceeded the capacity for sustainable wild root harvesting, effective cultivation ofall Echinacea species is imperative. Growers of E. angustifolia must take an interestin genetic preservation to protect their native sources of germplasm diversity from theconsequences of urban development, herbicide use and overgrazing (Price-Hurlburt,2000). Morphological races from a range of wild E. angustifolia seed sources havebeen identified in a common garden based on significant variation in aerial yield,height, seed survival, lodging/disease, growth habit, flowering and seed maturity(Little, 1999). Genetic variation within and among natural populations of E. angusti-folia is reportedly extremely high (Feghahati and Reese, 1994). Viles and Reese(1996) suggested that such genetic variability was responsible in part for reportedphytochemical variability (Bauer and Wagner, 1991). As a measure of phytochemicaldiversity among wild seed sources, Viles and Reese (1996) studied allelochemicalactivity in a greenhouse study, with the conclusion that natural selection was actingto differentiate wild populations of E. angustifolia genetically, through shiftingenvironmental pressures such as herbivory.
Cultivation of E. purpurea (L.) Moench has already enjoyed significant increases
through traditional selective breeding practices worldwide. Morphologically superiorE. purpurea lines from germplasm with demonstrated high genetic variability (Baumet al., 1999) resulted in doubled average phytochemical content in each of the majorchemical classes when compared to non-selected lines (Letchamo et al., 1998). Simi-lar selection of superior cultivars by phytochemical prediction would enhance E.
angustifolia cultivation.
We demonstrated pathway induction for the Echinacea secondary phytochemicals,
alkamides and ketoalken/ynes, using a naturally-occurring chemical mediator in E.
pallida (Binns et al., 2001). This phenomenon may be a mechanism under environ-mental control, which effectively minimizes the energetic costs of defense. However,there are no reports of genetic adaptation leading to differences in plant secondaryphytochemical metabolism, although the ability to direct resources to primary metab-olism (growth and differentiation) may evolve through stable genetic polymorphisms
S.E. Binns et al. / Biochemical Systematics and Ecology 30 (2002) 837–854
in plant species with a broad range of habitats and selection pressures (Herms andMattson, 1992).
The objective of the current study was quantitative evaluation of the genetic
component in phytochemical diversity of E. angustifolia populations from differentgeographic areas. We have evaluated the quantity and type of phytochemicalsaccumulated in young E. angustifolia roots from a range of wild populations, grownunder uniform environmental conditions. We also explored the association betweenthe observed phytochemical variability and latitude of germplasm sources.
2. Methods
2.1. Achene germination
Achenes from nine native populations of E. angustifolia were harvested from
August to October 1997 and 1998, then stored in a freezer at ⫺20 °C. Voucherspecimens are deposited at the Department of Agriculture Ontario Herbarium, codeDAO (Table 1). Botanical identification of source plants was performed in the fieldaccording to McGregor (1968) and confirmed in a concurrent morphometric taxo-nomic revision (Binns et al., in press), where only one population (no. 6) exhibitedintrogressant morphological characteristics (Table 1). Under sterile conditions, 50achenes from each accession were placed in a sealed container with holes (smallerdiameter than achenes, approx. 1 mm) and soaked in Plant Preservation Mixture(PPM) (10 ml/l in dH O) for 24 h on a rotary shaker. They were rinsed three
times with dH O and placed in petri dishes on filter paper (Whatman no. 1) soaked
with 1 ml/l ethylene (Ethrel) in distilled H O. Petri dishes were sealed with par-
afilm and placed in a growth chamber under constant light (5 µM/m2/s, incandescent)
Table 1Location, latitude, accession labels (corresponding to voucher specimens, coll. S. Binns, DAO) and num-ber of individuals for nine populations of Echinacea angustifolia
No. plants (n)
OK: Carter Co, Fox
OK: Carter Co, Alma
KS: Pottawatomie Co
NE: Richardson Co
a Introgressant characteristics from possible past hybridization between E. pallida X E. angustifolia
according to morphometic taxonomic analyses (Binns et al., in press).
S.E. Binns et al. / Biochemical Systematics and Ecology 30 (2002) 837–854
at 4 °C for 14 days. Gradually, over 5 days, the temperature was raised to 25 °Cand germination occurred within 10 days following temperature increase.
Mature germlings were potted in a mix of 5:3:1 vermiculite: Promix: quartz sand
(large grains) in cell packs for several weeks in the growth chamber (25 °C and 16h days) and eventually transplanted into individual 3 in. pots in a greenhouse (25
°C and 16 h days). The final sample size per population (Table 1) varied accordingto survival, which was between 21–78%. We used a completely randomized designon a single greenhouse bench.
2.2. Extraction and isolation
Fresh roots were harvested at the age of 6 months, washed thoroughly and covered
with 60% EtOH. They were coarsely chopped in a blender (Osterizer), followedimmediately by 60 s on the medium–high setting of a Polytron (Brinkmann Instru-ments, Westbury, NY) to completely release cell contents into solution. The root–ethanol slurry was shaken at medium speed (70 rpm) for 20 h, and filtered byBuchner filter (Whatman no. 1). Extracted root material was dried in an oven (50
°C), then weighed and discarded. The filtrate was rotary evaporated to dryness andre-dissolved in fresh 60% EtOH with the appropriate volume to achieve standardized0.5g/ml extracts. These were filtered for HPLC using 0.2 µm nylon membranes.
A validated method was used for HPLC phytochemical separations (Bergeron et
al., 2000). Hydrophilic chromatography was achieved using a solvent system of ace-tonitrile: 50 mM NaH PO pH 2.95, at a flow rate of 1.5 ml/min following a linear
gradient of 5–25% acetonitrile over 7 min. Lipophilic chromatography was achievedusing a solvent system of acetonitrile:water, at a flow rate of 1.0 ml/min followinga linear gradient of 40–80% acetonitrile over 15 min. In both systems, 5 µl of samplewas injected on a 7.5×4.6 mm C-18 column (3 µm particle size) (Lichrospher, MerckBDH Toronto, Canada). Lipophilic compounds were detected at 210 and 260 nmand hydrophilic compounds were detected at 326 nm.
2.3. Identification and quantitation
Reference standards of the first 11 compounds were obtained through isolation in
our laboratory or purchase as follows: undeca-2E,4Z-diene-8,10-diynoic acid isobu-
tylamide 1, dodeca-2E,4E,8Z,10E/Z-tetraenoic acid isobutylamide (tetraenes) 2+3,
pentadeca-2E,9Z-diene-12,14-diynoic acid isobutylamide 5, 2,3-O-dicaffeoyltartaric
acid (cichoric acid) 6 and echinacoside 7 were isolated by column chromatography
on silica gel and assessed for purity by 1H and 13C NMR spectral data (Bergeron et
al., 2000). Two others compounds were isolated by the same method (Bergeron et
al., unpublished): dodeca-2E,4E-dienoic acid isobutylamide 4 (by online UV spectra;
Bauer and Remiger, 1989) and cynarin 10 (by 1H and 13C NMR, Cheminat et al.,
1988). We purchased caftaric acid 9 (Dalton Chemical Laboratories Inc., Toronto,
Canada), and both caffeic acid 8 and chlorogenic acid 11 (Sigma Aldrich, St. Louis,
USA). We followed the method of other researchers (Perry, 1997), and identified
compounds 12–28 by their relative retention time to the marker compounds dodeca-
S.E. Binns et al. / Biochemical Systematics and Ecology 30 (2002) 837–854
2E,4E,8Z,10E/Z-tetraenoic acid isobutylamides 2+3, and online photodiode array UV
spectra (c.f. Bauer and Remiger, 1989), since they are not available for purchase
and isolation of adequate quantities has proven costly.
We calculated a response factor from the standard curve of each of the compounds
1–11, and used the response factor for tetraenes 2+3 to quantify compounds 12–28
in tetraene equivalents, which was acceptable for the purpose of mean quantitative
comparisons within and between populations in the present study. For each eluted
compound in experimental samples, we multiplied peak area by the appropriate
response factor to give µg compound/ml extract, then divided by the original concen-
tration of 0.5g extracted dried root/ml sample and multiplied by 103 to reach mg/g
dry wt.
2.4. Statistical analyses
The null hypothesis that there was no significant difference in phytochemical quan-
tity between populations (nine locations) was tested for the 26 phytochemicals singlyand collectively in 115 individual plants, as follows. The effect of each individualphytochemical on variation between populations was assessed by one-way ANOVAusing Systat software version 7.0 (SPSS Inc., Chicago, IL, 1999). Tukey's pairwisedifferences between the nine populations were also calculated for each individualphytochemical. Then, canonical discriminant analysis of the 26 phytochemicals usingSAS CANDISC procedure (SAS Institute Inc., Cary, NC, 1999) assessed squaredMahalanobis distances between populations, measured the variation within andbetween populations, and ascertained which phytochemical(s) contributed the mosttowards that variation (Sneath and Sokal, 1973). The resulting matrix of Mahalanobisdistances was subjected to principal coordinate analysis and single linkage clusteringusing NT-SYS-pc software (Rohlf (1999)) to provide several graphical represen-tations of the relative distances between the centroids of each population.
The relationship between geographical latitude and phytochemical content was
determined using simple linear regression (Systat v.7) of the population means foreach phytochemical (one at a time) on the latitude of the corresponding germplasmsource (in Table 1). When assumptions of normality and homoscedasticity were viol-ated, we used either the logarithmic transformation or weighting to correct for differ-ent variances and sample sizes (n) between populations. The justified weighting pro-cedure, in this particular program, consisted of multiplication of the population means(log-transformed) by the inverse of sample variance so that means with smaller vari-ances received more weight in the analysis.
3. Results
The mean levels of 28 constituents in representative samples from nine different E.
angustifolia populations are presented in Figs. 1–5. There was significant quantitativevariation (ANOVA p⬍0.05) between the mean levels of several lipophilic and hydro-philic compounds across all nine populations (Table 2).
S.E. Binns et al. / Biochemical Systematics and Ecology 30 (2002) 837–854
All mean accumulations of phenolics were significantly different by ANOVA
between the nine populations, except for caffeic 8 and chlorogenic 11 acids. Among
those compounds with significantly-different means (Table 2), the maximum mean
accumulations of cichoric acid 6 were observed in no. 9, of echinacoside 7 in no.
3, of caftaric acid 9 in no. 6, and cynarin 10 in no. 8 (Fig. 1).
Populations nos. 8 and 9 contained the maximum mean concentration of the fol-
lowing alkamides: 4, 5, 13, 14, 15, 16, 17, 18, 19, and each of those was significantly
S.E. Binns et al. / Biochemical Systematics and Ecology 30 (2002) 837–854
S.E. Binns et al. / Biochemical Systematics and Ecology 30 (2002) 837–854
Caffeic acid derivatives 6, 7, 8, 9, 10, 11 in E. angustifolia populations from Table 1 (mean
mg/g dwt±SEM).
different across all populations according to ANOVA (Table 2). The major alkam-
ides, 2+3 were found in similar quantity among all populations, except no. 7 (not
significantly different). Also, ANOVA of the mean differences in ketoalkene/ynes
across all populations was not statistically significant, although 20 and 21 were high-
est in population no. 7 and compound 22 was unique to nos. 3, 5 and 6.
3.1. Regression
A positive relationship between increasing phytochemical quantity and increasing
latitude (of parental populations) was statistically significant for the phenolics 6 and
7, and alkamide 14 (p⬍0.05, Table 3). However, there was a significant inverse
S.E. Binns et al. / Biochemical Systematics and Ecology 30 (2002) 837–854
Alkamides 1, 2+3, 4, 5, 12, 13 in E. angustifolia populations from Table 1 (mean mg/g
relationship between the quantity of phenolic 9 and alkamide 19 with increasing
latitude (Table 3).
3.2. Canonical discriminant analysis
A canonical discriminant analysis is considered acceptable when at least 75% of
the variation is explained by the three axes (Sneath and Sokal, 1973). Here, the firstthree canonical axes explain 79% of the phytochemical variation between popu-lations. An F-statistic of the squared Mahalanobis distances between population cen-troids (Fig. 6) determined that all were significantly distant from one another atp⬍0.001 or p⬍0.01 except the following pairs of populations; nos. 1 and 2(p=0.7850), nos. 1 and 5 (p=0.1914), nos. 2 and 4 (p=0.4075) and nos. 2 and 5(p=0.2553). Population no. 6 was most easily distinguished from the others. The
S.E. Binns et al. / Biochemical Systematics and Ecology 30 (2002) 837–854
Alkamides 14, 15, 16, 17, 18, 19 in E. angustifolia populations from Table 1 (mean mg/g
centroid of population no. 8 was also significantly segregated from other populations(p⬍0.0001), and even from its closest neighbour, no. 9 (p=0.0026). Fig. 7 is a threedimensional representation of the principle coordinates for the variation of eachpopulation along the first three canonical axes, which facilitates the visualization ofquantitative differences between the multivariate population means.
In the current study of wild E. angustifolia germplasm, quantitative phytochemical
production varied as a function of geographical distance between nine populations.
S.E. Binns et al. / Biochemical Systematics and Ecology 30 (2002) 837–854
Alkamides 23, 24+25, 26, 27, 28 in E. angustifolia populations from Table 1 (meanmg/g
Population nos. 3, 6, 7, 8 and 9 were the most significantly different according toseveral statistical analyses. Compared to the above, much smaller geographical dis-tances separated populations nos. 1, 2, 4 and 5 (Table 1), and these same populationswere the least distinguishable by ordination.
The most distinct chemotype was population no. 6 according to the multivariate
statistical results (Figs. 6 and 7). Population no. 6 contained only trace amounts of
E. angustifolia species markers, cynarin 10 and alkamides 17, 18, 23, but a large
amount of ketone 22 (a commercial E. pallida marker) and echinacoside 7 (which
was reported from both species in Bauer and Wagner, 1991; Binns, 2001). Consider-
ing both its morphological characteristics (Binns et al., in press) and its phytochem-
ical profile presented here, the chemotype of population no. 6 may be a result of
hybridization/introgression between E. pallida (Nutt.) Nutt. and E. angustifolia DC.
S.E. Binns et al. / Biochemical Systematics and Ecology 30 (2002) 837–854
Ketoalkene/ynes 20, 21, 22 in E. angustifolia populations from Table 1 (mean mg/g dwt±SEM).
Population no. 3 also slightly resembled E. pallida root profiles (Bauer et al., 1988)
with an unusual level of 22, as did no. 7 with the highest levels of 20 and 21.
However, there was no morphological or ecological indication that populations nos.
3 and 7 were possible hybrids or introgressants, and the phytochemical evidence
may be due to the young age of the plants in the current study.
Plant developmental stage influences secondary metabolism; defense compounds
are generally more concentrated and diverse when plants are young and more "appar-ent" to herbivores, but they are known to decrease with age as structural defensesare developed (Feeny, 1976). Therefore, as roots in the present study were stillyoung, and they yielded a greater variety of chemical defenses than what is reportedfor older commercial E. angustifolia roots (typically ⬎2 years) (Bauer and Wagner,1991), this might explain why populations nos. 3 and 7 had intermediate profiles.
It is useful to determine which constituents may be responsible for most of the
differences between potential chemotypes in a multivariate analysis. In the presentstudy, phytochemical variables that contributed the most to the squared Mahalanobis
S.E. Binns et al. / Biochemical Systematics and Ecology 30 (2002) 837–854
S.E. Binns et al. / Biochemical Systematics and Ecology 30 (2002) 837–854
Table 3Simple or weighted regression of the mean phytochemical quantities per population versus latitude ofgermplasm source for those compounds found to be significantly different by ANOVA (Table 2)
r2 (slope)
a LOGmean=mean transformed by the logarithm base 10.
b LOGVAR⫺1=inverse variance of the LOGmean.
c (-)=no results where normality assumption was violated in all trials.
distances between populations in the canonical discriminant analysis were alkamides
4∗, 5∗∗, 14∗, 19∗, and cichoric acid 6∗∗, echinacoside 7∗∗, and caftaric acid 9∗
(F-statistic probabilities ∗p⬍0.0001, ∗∗p⬍0.05). Both alkamide 18, and echinacoside
7 are currently used for commercial standardization of E. angustifolia, so their sig-
nificant variation as chemotype determinants is economically significant. Interest-
ingly, the root concentrations of five of those same compounds, 6, 7, 9, 14, and 19
varied significantly in relation to latitudinal variation (Table 3). Populations nos. 8
and 9 are potentially superior chemotypes with significantly increased concentrations
of compounds 6, 7 and 14 at higher latitudes and including the overall increasing
latitudinal trends of most alkamides and phenolics (Figs. 1–5). As well, we caution
that the significant inverse relationship of 9 and 19 with increasing latitude may be
an artifact of the regression, because there were no matching trends in the mean
population data (Figs. 1 and 4).
Latitudinal and quantitative variation of cichoric acid 6 and echinacoside 7 prom-
ises to be useful. Echinacoside 7 has been reported from all varieties and species of
Echinacea, except E. purpurea (Bauer and Wagner, 1991; Binns, 2001). Here, we
indicated that seed source within a single species (and the effects of latitude on that
location) influences the overall levels of echinacoside 7 in plant materials used for
herbal product manufacturing. It is therefore misleading to standardize Echinacea
products by % echinacoside concentration, which is often listed on herbal product
labels.
S.E. Binns et al. / Biochemical Systematics and Ecology 30 (2002) 837–854
Single linkage phenogram of the squared Mahalanobis distances between centroids of E. angusti-
folia populations numbered as in Table 1.
Immunostimulant activity was reported for cichoric acid 6, although it was not
measurable for caftaric acid 9 in tests of phagocytosis induction (Bauer, 1998). Cich-
oric acid 6 is the most abundant constituent in the flowers of all Echinacea species
(Bauer, 1998; Binns, 2001) with the highest quantity in young E. purprea flower
buds (Letchamo et al., 1998). In addition, E. purpurea roots contain 6 in large
amounts (Bauer and Wagner, 1991; Bauer, 1998; Binns, 2001) compared to much
smaller trace amounts in E. pallida and E. angustifolia roots where the major com-
pounds were echinacoside 7 and cynarin 10 (see Results; Binns, 2001). Therefore,
demonstrated latitudinal influence on cichoric acid accumulation in the roots of wild
E. angustifolia accessions, while not as quantitatively significant for this particular
S.E. Binns et al. / Biochemical Systematics and Ecology 30 (2002) 837–854
Principal coordinates of nine E. angustifolia populations representing the variation on the first
three canonical axes superimposed by a minimum spanning tree of the squared Mahalanobis distances(dashed lines).
species, has implications for potentially high-yielding Echinacea cultivar develop-ment, especially if the same latitudinal variation can be determined among cultivatedseed sources for E. purpurea.
Finally, the significant quantitative variation in E. angustifolia populations (see
Table 2) with respect to caftaric acid 9 and cynarin 10 may impact the Echinacea
products derived from different seed sources, once the pharmacological activities of
these pure compounds are investigated.
Increased phytochemical production in northern plant populations has been
reported for the phenolic DIMBOA in corn (Zea mays L.; Levin and York (1978)).
This research measured higher levels of DIMBOA from temperate germplasmsources compared to the tropical landraces. High levels of DIMBOA were associatedwith increased insect resistance in temperate varieties of corn (Xie (1991)). Similarly,in the present study, ecological gradients that exist across a latitudinal cline helpedto create germplasm heterogeneity in wild E. angustifolia populations which affectedthe phytochemical accumulations of both phenolics and alkamides in a controlledenvironment. These phenomena are best explained by stable or impermanent geneticpolymorphisms among populations of the widespread species E. angustifolia inresponse to selection pressures, such as habitat, competition and herbivory.
Those chemotypes which were most distinct in the current study may be identified
by their US county names: no. 3=Comanche (OK); no. 6=Cowley (KS); no. 7=Potta-watomie (KS); no. 8=Richardson (NE); and no. 9=Clay (IA) (Table 1). This infor-mation is particularly useful when combined with traditional breeding studies fordesirable morphology and disease resistance to improve cultivation of E. angustifoliaand thereby help to insure germplasm conservation. To provide tools for the improve-ment of modern phytomedicines, future investigations should target the effect of
S.E. Binns et al. / Biochemical Systematics and Ecology 30 (2002) 837–854
genetically-variant wild and cultivated sources of all Echinacea species and varietieson biologically-active phytochemical production.
The authors gratefully acknowledge the contribution of Echinacea angustifolia
achenes by Mark Widrelechner of NCRPIS, USDA. Funding for the research wasprovided by the Natural Science and Engineering Research Council of Canada, aswell as by Trout Lake Farm LLC, WA and the matching initiative fund of Agricul-ture Canada.
Bauer, R., 1998. Echinacea: Biological effects and active principles. In: Lawson, L.D., Bauer, R. (Eds.),
Phytomedicines of Europe: Chemistry and Biological Activity. American Chemical Society Sym-posium Series 691. American Chemical Society, Washington D.C, pp. 140–157.
Bauer, R., Remiger, P., 1989. TLC and HPLC analysis of alkamides in Echinacea drugs. Planta Medica
55, 367–371.
Bauer, R., Wagner, H., 1991. Echinacea species as potential immunostimulatory drugs. In: Wagner, H.,
Farnsworth, N. (Eds.), Economic and Medicinal Plant Research. Academic Press, London, pp. 253–321.
Bauer, R., Kahn, I.A., Wagner, H., 1988. TLC and HPLC analysis of Echinacea pallida and Echinacea
angustifolia roots. Planta Medica 54, 426–430.
Baum, B.R., Binns, S.E., Mechanda, S., Arnason, J.T., 1999. The Echinacea germplasm enhancement
project. In: Proceedings of the 1999 International Echinacea Symposium, Jun 3–5, Kansas City, MO.
Bergeron, C., Livesey, J.F., Awang, D.V.C., Arnason, J.T., Baum, B.R., Letchamo, W., 2000. A quantitat-
ive HPLC method for identity and quality assurance of Echinacea products on the North Americanmarket. Phytochemical Analysis 11, 1–9.
Binns, S.E., 2001. The taxonomy, phytochemistry and biological activity of the genus Echinacea
(Asteraceae). PhD. Dissertation. University of Ottawa, Ottawa, ON.
Binns, S.E., Inparajah, I., Baum, B.R., Arnason, J.T., 2001. Methyl jasmonate increases reported alkamides
and keto-alkene/ynes in Echinacea pallida (Asteraceae). Phytochemistry 57, 417–420.
Binns, S.E., Baum B.R., Arnason J.T. A taxonomic revision of the genus Echinacea (Heliantheae:
Asteraceae). Systematic Botany (in press).
Brevoort, P., 1998. The US botanical market: a new overview. Herbalgram 44, 33–48.
Cheminat, A., Zawatzky, R., Becker, H., Brouillard, R., 1988. Caffeoyl conjugates from Echinacea spec-
ies: Structures and biological activity. Phytochemistry 27, 2787–2794.
Feeny, P., 1976. Plant apparency and chemical defense. In: Wallace, J.W., Mansell, R.L. (Eds.), Biochemi-
cal Interactions between Plants and Insects. Recent Advances in Phytochemistry, vol. 10. Plenum,NY, pp. 1–40.
Feghahati, S.M.J., Reese, R.N., 1994. Genetic variation among and between Echinacea angustifolia popu-
lations. Agron Abstract p. 213.
Herms, D.A., Mattson, W.J., 1992. The dilemma of plants: to grow or defend. The Quarterly Review of
Biology 67 (3), 283–335.
Kindscher, K., 1989. Ethnobotany of Purple Coneflower (Echinacea angustifolia, Asteraceae) and other
Echinacea species. Econ. Bot 43 (4), 498–507.
Letchamo, W., Livesey, J.F. , Arnason, J.T., Bergeron, C., Krutilina, V.S., 1998. Cichoric acid and isobu-
tylamide content in Echinacea purpurea as influenced by flower developmental stages. Association for
S.E. Binns et al. / Biochemical Systematics and Ecology 30 (2002) 837–854
the Advancement of Industrial Crops (AAIC). New Crops and New Uses Biodiversity and AgriculturalSustainability, Nov 8–11, Phoenix, AZ
Levin, D.A., York, B.M., 1978. The toxicity of plant alkaloids: an ecogeographic perspective. Biochem.
Syst. Ecol 6, 61–76.
Little, R.S., 1999. Echinacea angustifolia: variation of agronomic and morphological traits. In: Proceed-
ings of the 1999 International Echinacea Symposium; Jun 3–5; Kansas City, MO.
McGregor, R.L., 1968. The taxonomy of the genus Echinacea (Compositae). Univ. Kansas Sci. Bull 48,
Perry, N.B., van Klink, J.W., Burgess, E.J., Parmenter, G.A., 1997. Alkamide levels in Echinacea purpu-
rea: a rapid analytical method revealing differences among roots, rhizomes, stems, leaves and flowers.
Planta Medica 63, 58–62.
Price-Hurlburt D., 2000. Population ecology of Echinacea angustifolia in central Kansas. PhD. Thesis.
University of Kansas, Lawrence
Rohlf, F.J., 1999. NTSYSpc: Numerical taxonomy and multivariate analysis system. SUNY, New York.
Shemluck, M., 1982. Medicinal and other uses of the Compositae by Indians in the United States and
Canada. Journal of Ethnopharmacology 5, 303–358.
Sneath, P.H., Sokal, R.R., 1973. Numerical Taxonomy. W. H. Freeman and Co, San Francisco, USA.
Viles, A.L., Reese, R.N., 1996. Allelopathic potential of Echinacea angustifolia DC. Env. Exp. Bot 36
(1), 39–43.
Xie, Y., 1991. Role of hydroxamic acids in the resistance of maize, Zea mays L., to western corn root-
worm, Diabrotica virgifera virfigera LeConte. Ph.D. Dissertation. University of Ottawa, Ottawa, ON.
Source: http://echinacea.umn.edu/summer/binns&al2002a.pdf
IMC-Jordan started the technical preparations to host the annual conference of the Interna-tional Council of Management Consulting Insti-tutes (ICMCI) slated next September. Participants from 48 different countries are ex-pected, as the Conference will be an important working meeting for all to attend. A good por-tion of the agenda will be devoted to the ICMCI Breakthrough Strategy Initiative to sharpen its direction and determine the next steps. Another focus is the professional Standards Committee
ACMG STANDARDS AND GUIDELINES ©American College of Medical Genetics and Genomics laboratory testing of CYP2D6 alleles in relation to tamoxifen therapy Elaine Lyon, PhD, FACMG1, Julie Gastier Foster, PhD, FACMG2,3, Glenn E. Palomaki, PhD4, Victoria M. Pratt, PhD, FACMG5, Kristen Reynolds, PhD6, M. Fernanda Sábato, MS7,