Estrogen and cancer
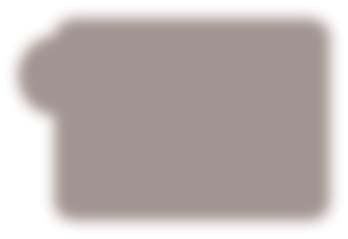



Estrogen and Cancer
Jing Liang1,2 and Yongfeng Shang1,2,∗
Tianjin Key Laboratory of Medical Epigenetics, Department of Biochemistry and Molecular
Biology, Tianjin Medical University, Tianjin 300070, China; email: [email protected]
2Key Laboratory of Carcinogenesis and Translational Research (Ministry of Education),
Department of Biochemistry and Molecular Biology, Peking University Health Science Center,Beijing 100191, China; email: [email protected]
by Harvard University on 02/12/13. For personal use only.
Annu. Rev. Physiol. 2013. 75:225–40
Annu. Rev. Physiol. 2013.75:225-240. Downloaded from www.annualreviews.org
First published online as a Review in Advance on
estrogen receptor, breast cancer, tamoxifen, endocrine resistance
The Annual Review of Physiology is online at
Estrogen exhibits a broad spectrum of physiological functions ranging from
This article's doi:
regulation of the menstrual cycle and reproduction to modulation of bone
density, brain function, and cholesterol mobilization. Despite the beneficial
2013 by Annual Reviews.
actions of endogenous estrogen, sustained exposure to exogenous estrogen is
All rights reserved
a well-established risk factor for various cancers. We summarize our current
∗Corresponding author
understanding of the molecular mechanisms of estrogen signaling in normaland cancer cells and discuss the major challenges to existing antiestrogentherapies.
ESTROGEN METABOLISM IN NORMAL AND CANCER CELLS
Estrogen sex steroid hormones exhibit a broad spectrum of physiological functions ranging from
regulation of the menstrual cycle and reproduction to modulation of bone density, brain function,
and cholesterol mobilization (1–3). Despite the normal and beneficial physiological actions of
endogenous estrogen in women, abnormally high estrogen levels are associated with the increased
incidence of certain types of cancer, especially those of the breast and endometrium. In 1991, the
stimulating hormone
Women's Health Initiative (WHI) research program initiated a 15-year study aimed at evaluating
the beneficial effects of postmenopausal hormone replacement therapy (HRT) on heart diseases,
bone fractures, and cancers; 161,808 generally healthy women of ages 50–79 were enrolled in this
study. The WHI was terminated prematurely in 2002 due to the increased incidence of breast
cancer, stroke, and cardiovascular complications in women treated with estrogen alone or with
a combination of estrogen and progestogen (4). Shortly afterward, the US National Toxicology
Program made the unprecedented decision to classify estrogens as carcinogens. The InternationalAgency for Research on Cancer (IARC) also listed both estrogen and estrogen-progestogen-combined postmenopausal therapies as known human carcinogens. In this review, we focus onthe mechanisms of estrogen action in carcinogenesis and discuss current antiestrogen strategiesin cancer treatment. Due to space constraints, we apologize in advance to the many outstandingresearchers whose work we cannot cite.
The predominant intracellular estrogen is 17β-estradiol (E2). Other types of estrogen include
estrone (E1) and estriol (E3) (Figure 1a). In premenopausal women, E1 and E2 are secreted
primarily by the ovaries during the menstrual cycle, with minor levels derived from adipose tis-
sue and the adrenal glands. The placenta also produces E3 during pregnancy. In the ovaries,
granulosa cells synthesize estrogen from androgen (5). Ovarian production of estrogen is regu-
lated by the hypothalamic-pituitary-ovarian (HPO) axis and begins by anterior pituitary release
of luteinizing hormone (LH) and follicle-stimulating hormone (FSH) in response to the hy-
pothalamic peptide gonadotropin-releasing hormone (GnRH). Acting in concert, LH stimulates
androgen production, whereas FSH upregulates aromatase, which catalyzes the rate-limiting and
final step of estrogen biosynthesis: the aromatization of androgen to estrogen. During ovula-
tion, E2 production rises dramatically by eight- to tenfold. High levels of estrogen in turn act
via negative feedback to dampen estrogen production to inhibit the release of GnRH, LH, and
FSH (6).
The primary mediator of estrogen biosynthesis in postmenopausal women is aromatase, which
by Harvard University on 02/12/13. For personal use only.
is found in adipose tissue as well as in the ovaries, placenta, bone, skin, and brain (7, 8). Aftermenopause, ovarian estrogen biosynthesis is minimal, and circulating estrogen is derived prin-cipally from peripheral aromatization of adrenal androgen. As such, for obese postmenopausal
Annu. Rev. Physiol. 2013.75:225-240. Downloaded from www.annualreviews.org
women, adipose tissue becomes the main source of estrogen biosynthesis; this biosynthetic routeis far less significant for nonobese postmenopausal women (9). Although aromatase is critical tomaintaining normal estrogen levels in both premenopausal and postmenopausal women, thesegroups respond differently to aromatase inhibitors (AIs), which are used as antiestrogen therapyto treat breast cancer. In premenopausal women, the HPO axis is functional, with estrogen levelschanging dramatically throughout the menstrual cycle. As a result, the effects of AIs are quicklyoverridden when estrogen biosynthesis is driven by the next cycle of GnRH, LH, and FSH secre-tion. Thus, AIs are much more efficacious in postmenopausal women, whose HPO axis is no longeractive. In men, the testes also produce significant amounts of aromatase, allowing for androgenaromatization and estrogen synthesis (10).
Numerous studies have demonstrated the association of estrogen with the development and/or
progression of various types of cancer, including cancers of the breast, endometrium, ovary,
Liang · Shang
(a) The chemical structures of estrogens and selective estrogen receptor modulators. (b) The functional domains of estrogen receptor(ER). Both ERα and ERβ possess six distinct domains (A–F). The N-terminal A/B domain encodes a hormone-independenttranscriptional activation function (AF-1). The AF-1 domain is responsible for the major functional difference between ERα and ERβ.
Domain C corresponds to the highly conserved DNA-binding domain (DBD). Region D, the hinge region, separates the DBD and theligand-binding domain (LBD). Region E encodes the LBD, which is located close to the C-terminal portion. Regions E and F alsoharbor a second transcriptional activation function domain (AF-2), which activates transcription in response to estrogen.
prostate, lung, and colon (3, 11). Estrogen is a classical etiological factor for breast cancer and
by Harvard University on 02/12/13. For personal use only.
endometrial cancer, and most of our current knowledge of estrogen action comes from clinicaland laboratory studies of these two types of cancer.
Annu. Rev. Physiol. 2013.75:225-240. Downloaded from www.annualreviews.org
MOLECULAR MECHANISMS OF ESTROGEN ACTION
Estrogen mediates its biological effects in target tissues primarily by binding to specific intracellularreceptors, estrogen receptor (ER)α and ERβ. Since the initial reports of ER more than 50 yearsago (12), tremendous efforts have been made to decipher the complex molecular mechanisms ofestrogen-ER action. In the classical model of estrogen-ER function, the complex acts as a ligand-activated transcription factor to regulate the expression of multiple target genes. An alternativeview is that estrogen exerts rapid, stimulatory effects on intracellular signal transduction pathways.
This nongenomic pathway begins outside the nucleus and is independent of gene transcription(13–15).
ERα and ERβ are encoded by ESR1 and ESR2, respectively; each gene is located on a different
chromosome. ERα and ERβ are widely expressed in many tissues, such as the uterus, ovary,
ER: estrogen receptor
mammary gland, prostate, lung, and brain. The expression levels and subtypes of ER are primary
www.annualreviews.org • Estrogen and Cancer
factors that determine tissue-specific estrogen responsiveness (16, 17). Both ERα and ERβ are
members of the nuclear hormone receptor superfamily of ligand-regulated transcription factors
that contain modular structures and discrete functional domains (Figure 1b). ERα and ERβ
are highly homologous (∼96%) in their DNA-binding domains (DBDs) and possess moderate(53%) sequence identity in their ligand-binding domains (LBDs). The major functional difference
between ERα and ERβ appears to be determined by the hormone-independent transcriptionalactivation function (AF-1) domains in their respective N termini (18). The pro-oncogenic effect
EREs: estrogen
response elements
of estrogen is mediated primarily by ERα activation of target genes that promote cell proliferationor decrease apoptosis.
The binding of estrogen to ERs induces conformational changes in protein structure that allow
receptor dimerization and interaction with coactivators. In some cases, the activated estrogen-ER
complex directly binds to estrogen response elements (EREs) in gene promoters, leading to
the transcriptional activation of target genes. Alternatively, the estrogen-ER complex indirectlyactivates gene transcription through protein-protein interactions with other transcription factorssuch as activator protein 1 (AP1), specificity protein 1 (SP1), nuclear factor-κB (NF-κB), cAMPresponse element–binding protein (CREB), runt-related transcription factor 1 (RUNX1), p53,and signal transduction and activator of transcription 5 (STAT5) (2, 19–22). Interaction withother transcription factors allows the estrogen-ER complex to regulate target genes whosepromoters do not harbor a bona fide ERE.
The relative abundance of ERα versus ERβ in a given cell type appears important for medi-
ating tissue-specific estrogen responsiveness (16). ERβ was discovered in 1996, almost 40 yearsafter the initial characterization of ERα (18). Genetic and pharmacological lesions directedagainst either ERα or ERβ show that these two estrogen nuclear receptors exert opposing effectson cell proliferation and apoptosis (23–26). Another variant of ERα, the truncated ERα-36,appears to mediate membrane-initiative effects of estrogen signaling, and as does ERβ, ERα-36interferes with ERα activity, as well as with antiestrogen treatment of breast cancer (27). A recentreview summarized various ER subtypes and their roles in cancer biology and therapy (16).
To gain full transcriptional regulatory activity, the estrogen-ER complex needs to recruit
diverse transcriptional coregulators, many of which have various enzymatic activities to modifychromatin structures or to interact with the general transcription apparatus. More than 300 pro-teins have been shown to interact with one or more members of the nuclear receptor superfamily,and many of these proteins also interact with ER (13, 28). The p160 family proteins (or SRCs),
by Harvard University on 02/12/13. For personal use only.
including three distinct but related homologous members—SRC-1, SRC-2 (GRIP1), and SRC-3(AIB1)—are the best-characterized coregulators of ER (29). SRCs facilitate a transcriptionallypermissive environment through direct and/or indirect recruitment of other coactivators that
Annu. Rev. Physiol. 2013.75:225-240. Downloaded from www.annualreviews.org
have chromatin-remodeling and histone modification activities. In addition, SRC proteins possessintrinsic but weak histone acetyltransferase (HAT) activity and mediate transcriptional activationby directly acetylating conserved lysine residues on histones and possibly other coregulators (30,31). Although all three SRCs directly interact with ER, they recruit different sets of secondarycofactors to further fine-tune the transcriptional regulatory activity of the ER-nucleated apparatus(32). The assembly and disassembly of the ER complex are extremely dynamic, and variation ingene expression among tissues under different conditions can greatly affect the final compositionof the ER complex.
ERs, as well as their coregulators, are subjected to a variety of posttranslational modifications
(PTMs), which further influence the stability, subcellular localization, transactivity, and hormonesensitivity of the ER-nucleated transcriptional apparatus. Approximately 22 sites throughoutERα are subjected to various modifications, including phosphorylation, methylation, acety-lation, sumoylation, and ubiquitination. For example, ER phosphorylation by several kinases,
Liang · Shang
such as mitogen-activated protein kinase (MAPK) and protein kinase A (PKA), enhances ERtranscriptional activity (33, 34); ER acetylation by the HAT p300 regulates ER transactivityand hormone sensitivity (35); and ER ubiquitination leads to ER degradation and thus reducesER bioavailability (36). A recent comprehensive review summarizes the different types of ERPTMs and their biological functions (37). Besides ER, various coregulators are also subjectedto PTMs. PTMs of coregulators change their protein conformation and greatly affect thesubunit composition, potency, and target selectivity of the final ER complex. For example,coactivator-associated arginine methyltransferase 1 (CARM1), a well-established coactivator ofERα and a type I protein arginine methyltransferase, catalyzes asymmetric dimethylation ofarginine residues (38). Substrates of CARM1 include histone H3 (39), the ER-interacting HATsCBP and p300 (40), the p160 family member AIB1 (41), and the C-terminal domain of RNApolymerase II (42). Accordingly, CARM1 regulates ERα-mediated gene transcription in multipleways from changing local chromatin structures to affecting the general transcription apparatusand specific cofactors. PKA phosphorylates CARM1. Whereas unphosphorylated CARM1 bindsto ERα in the classic ligand-dependent manner, phosphorylated CARM1 binds to the unligandedLBD of ERα and regulates the expression of different sets of genes (43).
Many genes involved in multiple intracellular pathways are directly regulated by the large
estrogen-ER transcription apparatus. Over the past several years, several groups have soughtto identify all ER target genes in the genome to fully understand the biological functions ofestrogen.
Prior to genomic approaches, a hypothesis-driven, candidate gene approach yielded some well-
characterized ER target genes, such as trefoil factor 1 (TFF-1, also known as pS2) and cathepsin D,
that have been used to delineate the molecular mechanisms of estrogen signaling (44). The rapid
advancement of array-based technology and next-generation sequencing has greatly facilitated the
identification of novel ER target genes. Data obtained by these technologies have transformed
our perception of the way that ER functions (Figure 2). In the conventional view, ligand-bound
ERs function at the promoter regions of target genes. Genome-wide mapping of ER-binding
sequences of human chromosomes 21 and 22 by using ChIP-tilling arrays indicates that the
majority of the ER-binding sites are distant (as far as several hundreds of kilobases away) from the
transcription start sites of regulatory genes and that these distal ER-binding domains often function
as transcriptional enhancers. Moreover, many of these binding sites lack a consensus ERE but are
also bound by Forkhead protein FoxA1, which is obligate for a genuine ER-chromatin interaction
by Harvard University on 02/12/13. For personal use only.
(45, 46). ChIA-PET (chromatin interaction analysis using paired-end tag sequencing) technology,which couples chromosome conformation capture with high-throughput sequencing, allows forthe global identification of three-dimensional chromatin loops formed in cells upon estrogen
Annu. Rev. Physiol. 2013.75:225-240. Downloaded from www.annualreviews.org
induction. Treating hormone-deprived MCF-7 breast cancer cells with estrogen for a short periodyields 689 ER-associated chromatin interaction complexes, which are made up of duplexes andmore complex interactions. By combining the ChIA-PET data with gene expression profiles,Fullwood et al. (47) discovered that a significant percentage of these clusters are transcriptionhot spots induced by estrogen. The actual anchor points in these clusters can be separated byhundreds of kilobases or even by megabases in linear two-dimensional chromatin, suggesting thatlong-range chromatin interactions between DNA elements play key roles in estrogen-inducedtranscriptional regulation (47).
Although ERα and ERβ have similar affinities for E2 and bind similar DNA response elements,
each receptor exhibits a unique genomic signature (45, 46, 48). Genomic profiling and ChIP-Seqstudies find that gene networks involved in cell proliferation are activated differently by these twoestrogen receptors, implying that a balance of ERα and ERβ signaling ultimately determines howestrogen acts.
www.annualreviews.org • Estrogen and Cancer
by Harvard University on 02/12/13. For personal use only.
Molecular mechanisms of estrogen-induced gene transcriptional regulation. E2 diffuses into the cell and binds to ER (ERα/ERβ).
Liganded receptors enter the nucleus and form homo- or heterodimeric complexes that bind to estrogen-response elements (EREs) onthe target gene promoters. Liganded receptors can also act as monomers and bind to target gene promoters through interaction withother transcription factors such as Sp1 and Ap1. Promoter-associated ERs initiate gene transcription through interaction with
Annu. Rev. Physiol. 2013.75:225-240. Downloaded from www.annualreviews.org
coregulators and the basal transcriptional machinery. Researchers recently found that ER can bind to enhancer elements far distallyfrom target genes regulated by ER. In some cases, the presence of the pioneer factor FoxA1 is necessary for ER to bind to its DNAresponse element.
ESTROGEN SIGNALING IN CARCINOGENESIS
Mechanistic studies have established a solid foundation for our understanding of estrogen signalingin carcinogenesis. Genetic changes associated with the oncogenic effects of estrogen match wellwith the classic genetic markers of human cancers. Indeed, the activated estrogen-ER complexregulates various genes that play key roles in cell proliferation and cell cycle progression. Themitogenic effects of estrogen are particularly clear at the G1-to-S transition, during which thekey effectors of estrogen action are c-Myc and cyclin D1 (49, 50). One of the earliest responsesto estrogen is increased c-Myc expression, which occurs within 15 min of estrogen stimulation.
Estrogen also rapidly induces cyclin D1 expression, and antiestrogen agents have a converse
Liang · Shang
acute, inhibitory effect. Inhibition of c-Myc or cyclin D1 activity abrogates estrogen-stimulatedbreast cancer cell proliferation, whereas induction of c-Myc or cyclin D1 can mimic the effectsof estrogen and reinitiate cell cycle progression in antiestrogen-arrested cells (51). Although thegenes encoding c-Myc and cyclin D1 have been well established as estrogen-induced genes formore than 20 years, the underlying molecular mechanisms of estrogen action on these two genesare still not fully understood. In the case of the c-Myc gene, it was originally proposed that anatypical estrogen-responsive region in the c-Myc proximal promoter is the cis element whereactivated ER binds and functions. However, recent studies indicate that cooperation betweenER and AP1 at a distal enhancer element upstream of the transcriptional start site of c-Myc isresponsible for estrogen-induced c-Myc expression (52). Unlike ERα, ERβ inhibits estrogen-induced cell proliferation. Forced expression of ERβ in ERα-positive breast cancer T47D cellsinhibits the proliferative responses to E2, possibly by suppressing the expression of cyclin E butnot that of cyclin D1 (53).
Estrogen inhibits apoptosis by upregulating antiapoptotic Bcl-2 and Bcl-XL expression in breast
cancer cells (54). The estrogen-ERα complex interacts with proteins such as c-Src through nonge-nomic action and activates the MAPK and PI3K/Akt pathways, which are classically linked to cellsurvival (19). Paradoxically, estrogen induces apoptosis under certain circumstances. Before theintroduction of tamoxifen, high-dose estrogen was used to induce tumor regression of hormone-dependent breast cancer in postmenopausal women. This regimen is of clinical interest, given thatlong-term treatment of breast cancer with antiestrogen tamoxifen often leads to drug resistanceand that sustained tamoxifen exposure may sensitize breast cancer cells to high-dose or even low-dose estrogen therapy (55). The mysterious dual effects of estrogen on apoptosis may be due toaltered global gene expression and to redirected intracellular signaling after long-term hormonedeprivation (56, 57).
The rapid growth and division of tumor cells require sufficient nutrient supply from blood via
angiogenesis. Angiogenesis involves several steps, including the degradation of existing vascularbasement membrane, the proliferation and migration of endothelial cells into tubular structuresin the tissue, and the formation of new matrix around neovessels. Estrogen induces blood vesselformation in the uterus during menstrual cycle. In breast cancer cells, E2 stimulates the secretionof interleukin 8 and vascular endothelial growth factor, both potent inducers of angiogenesis.
In contrast, antiestrogen tamoxifen decreases tumor angiogenesis in ER-positive breast cancer(58–60).
by Harvard University on 02/12/13. For personal use only.
Metastasis to distal organs is a major cause of death in cancer. In general, the E2-ERα path-
way does not stimulate tumor metastasis. ER-positive breast cancers have less metastasis potentialcompared with more invasive ER-negative breast cancers. In addition, ER-positive breast cancer
Annu. Rev. Physiol. 2013.75:225-240. Downloaded from www.annualreviews.org
patients often have a better prognosis than do ER-negative breast cancer patients. Epithelial-mesenchymal transition is now considered the first step in metastasis. E2-ERα signaling up-regulates epithelium-related transcription factors such as GATA3 and FOXA1 and promotesmammary epithelial differentiation along the luminal/epithelial lineage (61). Estrogen signalinginhibits de novo synthesis of RelB, a subunit of NF-κB that represses E-cadherin expression andpromotes cell migration and invasion (62). Moreover, forced expression of ERα in ERα-negativebreast cell lines induces the epithelial phenotype and reduces invasiveness, whereas knockdownof ERα in ERα-positive breast cell lines leads to the mesenchymal phenotype and increasedinvasiveness (63). Interestingly, the upregulation of several ER coregulators, such as AIB1 (am-plified in breast cancer 1), SRC-1, and PELP1 (proline, glutamate, and leucine-rich protein 1),promotes breast cancer invasiveness and metastasis (64). For example, AIB1 is encoded by awell-known oncogene that is often overexpressed in breast cancer. In addition to stimulating cellproliferation, AIB1 stimulates tumor cell motility and invasion. The Drosophila AIB1 homolog
www.annualreviews.org • Estrogen and Cancer
is crucial for ovarian border cell migration and invasion during oogenesis (65). Elevated AIB1expression is significantly correlated with seminal vesicle invasion and lymph node metastasis ofprostate cancer (66). AIB1 directly regulates the transcription of matrix metalloproteinase (MMP)-
2 and MMP-13 through its coactivation of AP1 and PEA3/ETV4 (ETS translocation variant 4)
(66). AIB1 can promote cancer cell motility while remodeling the local microenvironment tofacilitate tumor cell invasion into the adjacent microenvironment (67). Instead of interactingdirectly with the estrogen-ER complex, AIB1 is thought to participate in other signaling trans-duction pathways or to act as a coregulator of other transcription factors in promoting tumormetastasis (64).
INTERFERING WITH ESTROGEN ACTION IN CANCER TREATMENT
Antiestrogen strategies remain the primary breast cancer treatment because at least 70% of breastcancers are classified as ER-positive breast cancers. As such, modulation of estrogen signaling hasbeen a mainstay of breast cancer treatment for more than a century. Prior to the developmentof antiestrogen therapies, surgical oophorectomy was the chosen method of eliminating estrogenfunction in premenopausal breast cancer patients. Tamoxifen was introduced in the 1970s, fol-lowed by the rapid development of selective estrogen receptor modulators (SERMs) and AI. Withthe success of these antiestrogen drugs, the American Society of Clinical Oncology no longerrecommends ovarian ablation in systematic breast cancer therapy (68).
SERMs are a group of synthetic chemical compounds that are structurally related to estro-
gens and that bind to and modulate ER function in different tissues (2). The FDA has approved
three SERMs: raloxifene, toremifene, and tamoxifen (Figure 1a). Raloxifene, a second-generation
SERM, is used mainly to treat or prevent osteoporosis. Toremifene has antiestrogenic activity in
the mammary gland. It is presently used in postmenopausal women with metastatic breast cancer
(69). In 2009, the FDA approved toremifene to reduce fractures in men who had prostate cancer
and who were receiving androgen deprivation therapy.
Fulvestrant is representative of yet another type of ER modulator that promotes ER turnover
in cells (70, 71). Fulvestrant has been approved as a third-line treatment for ER-positive metastaticbreast cancer in postmenopausal women.
Tamoxifen was the first drug developed to target ER function and, due to its efficacy and
low price, remains the preferred choice for treating hormone-sensitive breast cancers. Although
by Harvard University on 02/12/13. For personal use only.
tamoxifen was initially used only in the treatment of advanced breast cancer in postmenopausalwomen, it is now used as an adjuvant therapy to reduce the risk of breast cancer recurrenceafter surgery in both pre- and postmenopausal women, as well as in women at high risk for
Annu. Rev. Physiol. 2013.75:225-240. Downloaded from www.annualreviews.org
breast cancer. A large STAR (Study of Tamoxifen and Raloxifene) clinical study compared theefficacies of tamoxifen and raloxifene in reducing the incidence of breast cancer. Finished in 2006,STAR concluded that raloxifene is as effective as tamoxifen, although there were statisticallyinsignificantly fewer uterine cancers and blood clots in women taking raloxifene (72). Tamoxifengreatly reduces the rate of breast cancer recurrence and has contributed significantly to the 25–30%decrease in breast cancer mortality in the past two decades (73).
Tamoxifen acts as an ER antagonist in breast cancer cells. Crystal structure studies indicate
that the ER LBD interaction surfaces are composed of amino acid residues belonging to α-helices3, 4, 5, and 12. When the ERα LBD is complexed with E2, α-helix 12 is positioned over theligand-binding pocket and forms an interaction surface for the recruitment of coactivators. Incontrast, when either the ERα LBD or the ERβ LBD is liganded with tamoxifen or raloxifene,α-helix 12 is displaced from its agonist position and occupies the hydrophobic groove formed byα-helices 3, 4, and 5, causing α-helix 12 to block the coactivator interaction surface (2, 74). Despite
Liang · Shang
acting as an ER antagonist in the breast, tamoxifen displays partial estrogenic effects in other targettissues, such as the bone, uterus, and cardiovascular system. These partial estrogenic actions havebeneficial effects on the bone and the cardiovascular system in postmenopausal women. However,
tamoxifen has been associated with an increased incidence of endometrial cancer. Differential
expression of ER coregulators may contribute to the tissue-specific response to tamoxifen (75).
failure to respond to
Comparison of gene expression profiles in cancerous versus normal endometrial epithelial cells
antiestrogen therapyin the treatment of
treated with tamoxifen indicates that PAX2, one of the PAX family of transcription factors, is
the key player in tamoxifen-stimulated endometrial cancer (76). Finally, because ERβ does not
stimulate breast or uterine tissues in response to E2, selective ERβ agonists may be beneficial in
manifestation of a
treating breast cancer (16, 17).
phenotype, via changes
Breast cancer tissues express aromatase and produce higher levels of estrogen than do non-
in chromatin states,
cancerous cells (7). Because aromatase catalyzes the final and rate-limiting step in estrogen biosyn-
that can be transmitted
thesis, inhibitors of this enzyme are an effective targeted therapy for breast cancer. As discussed
to the next generationof cells or individual
above, AIs are used mainly to treat breast cancer in postmenopausal women. AIs have also been
without altering the
combined with radiation therapy and/or chemotherapy to treat recurrent, metastatic, and high-
risk endometrial cancer. There are two types of AIs. Type I inhibitors have a steroid-like structure
sequence (genotype)
similar to that of androstenedione, the substrate of aromatase, and the covalent binding betweenthese inhibitors and aromatase irreversibly inhibits the enzyme. In the case of type II inhibitors (ornonsteroidal AIs), binding between the inhibitors and aromatase is noncovalent, and androgen cancompete with these agents and relieve the inhibitory effect on aromatase function. Three AIs—anastrozole, letrozole, and exemestane—are FDA approved and used in clinical practice. Amongthese agents, exemestane is a type I inhibitor, and anastrozole and letrozole are type II inhibitors.
AIs can be used after five years of tamoxifen treatment, as recommended by the National Compre-hensive Cancer Network breast cancer guidelines. AIs are often effective in tamoxifen-resistantbreast cancer (7) because AIs reduce estrogen biosynthesis, whereas tamoxifen antagonizes estro-gen action within breast cancer cells.
EPIGENETICS AND ENDOCRINE RESISTANCE IN CANCER
Although antiestrogen drugs have proven to be the most successful targeted cancer therapy inmodern medicine, drug resistance remains a major challenge in the treatment of breast cancer,even with positive ER expression. The National Cancer Institute estimates that more than 200,000
by Harvard University on 02/12/13. For personal use only.
Americans are diagnosed with breast cancer every year. More than 65% of breast tumors expressERα, but fewer than half of those tumors respond to antiestrogen therapy (77). Moreover, asignificant number of patients who initially respond to antiestrogen agents eventually become
Annu. Rev. Physiol. 2013.75:225-240. Downloaded from www.annualreviews.org
refractory and exhibit a more aggressive disease with a poorer prognosis. Numerous studies havetried to elucidate the underlying mechanisms of both intrinsic and acquired endocrine resistance,and various approaches have been proposed to enhance the antitumor efficacy of tamoxifen and AIs.
Fighting endocrine resistance remains a significant challenge in the field of breast cancer research.
Recently, several comprehensive reviews have focused on the possible underlying mechanisms ofendocrine resistance in breast cancer (19, 28, 78). Most research in this area has focused ontamoxifen resistance, given its widespread use and the existence of tamoxifen-resistant cell linesand animal models for mechanistic studies.
In general, resistance to antiestrogen therapy can be caused by genetic or epigenetic deregula-
tion of all components in the estrogen signaling pathway. The prodrug tamoxifen is first convertedto its active metabolite, endoxifen, by the hepatic drug-metabolizing enzyme cytochrome P4502D6 (CYP2D6). Polymorphisms in the CYP2D6 gene alter the efficiency of the tamoxifen-to-endoxifen conversion and can greatly affect the clinical response to tamoxifen. Although a strong
www.annualreviews.org • Estrogen and Cancer
link between CYP2D6 polymorphisms and tamoxifen response is unestablished, genetic screeningfor CYP2D6 variants may eventually prove useful for guiding the selection and dose of tamoxifen(79).
Ligand binding to ER recruits a panel of coactivators or corepressors; variation in coregulator
expression greatly affects the composition and output of the large ligand-ER complex. Conse-
quently, abnormal expression of ER coregulators, such as AIB1 and SRC-1, or of components ofother signaling pathways, such as ERBB2, can contribute to tamoxifen resistance (19, 80). Geneticmutation, gene amplification, gene fusion, and epigenetic deregulations can alter the expression ofcoregulators. For example, both AIB1 overexpression by gene amplification and certain AIB1 poly-morphisms cause tamoxifen resistance in patients (67, 81). Overexpression of the specific splicingvariant AIB3 can also affect responsiveness to antiestrogen therapy because this variant appearsto be more potent than wild-type AIB1 in provoking in vivo pathological changes in the mammarygland (67). Dysregulation of these ER targets is thought to oppose the antagonistic effects of tamox-ifen and to contribute to drug resistance. For example, overexpression of cyclin D1 or underexpres-sion of Bcl-2 is associated with many tamoxifen-resistant breast cancer patients (19). Large-scalegenomic studies have identified gene signatures that predict therapeutic responses to endocrinetherapies, albeit with inconsistent results (19). In addition, noncoding RNAs such as miR-451 mayalso play a role in tamoxifen resistance (82, 83). The studies suggest that targeting specific genesassociated with resistance may increase the efficacy of tamoxifen, as shown for trastuzumab, an anti-ERBB2 drug used as an adjuvant therapy in ER-positive, ERBB2-positive breast cancer (19, 28).
Efforts to define epigenetic mechanisms underlying endocrine resistance have been made (80,
84). Tamoxifen resistance emerges two to three years after the initial drug administration. Thistime factor suggests that defects in epigenetic marks, rather than primary DNA mutations, likelyplay a role in the progression of drug resistance. Epigenetic events can impact chromatin by di-rect DNA methylation at cytosine on CpG sequences, PTMs of histones, and the presence ofhistone variants and nonhistone chromosomal proteins. All these epigenetic factors greatly in-fluence local gene expression, and the reversibility of epigenetic modifications also makes themdruggable. Environmental cues influence epigenetic regulation, and it is tempting to speculatethat long-term treatment with tamoxifen or other antiestrogen agents may induce epigeneticchanges in breast cancer and endometrial cancer cells. Epigenetic factors may also contributeto endocrine resistance in breast cancer, with loss of ER expression attributed to promoter hy-permethylation of the ESR1 promoter (16). Treating ER-negative breast cancer cells with the
by Harvard University on 02/12/13. For personal use only.
DNA methyltransferase (DNMT) inhibitor AZA restores ER expression and sensitizes these cellsto tamoxifen treatment (85). ESR1 is not the only gene that is subjected to abnormal methyla-tion in tamoxifen-resistant breast cancer cells. Candidate gene and unbiased approaches using
Annu. Rev. Physiol. 2013.75:225-240. Downloaded from www.annualreviews.org
high-throughput DNA methylation profiling revealed different promoter methylation signaturespredictive of tamoxifen responsiveness (85, 86). Unfortunately, other than PITX2 (paired-likehomeodomain 2), a consensus of relevant markers affected by methylation has yet to emerge. Inthis respect, DNA methylation mapping by whole-genome bisulfite sequencing may be useful inidentifying the relevant markers of endocrine resistance.
Although histone modifications also affect global or individual gene expression, less is known
about how abnormal histone modifications contribute to endocrine resistance in breast cancer.
Lysine acetylation of histone H3 and H4 often leads to an open chromatin structure and there-fore correlates with transcriptional activation. Histone acetylation is catalyzed by HATs and isremoved by histone deacetylases (HDACs). Mutations or abnormal expression of several HATs,such as CBP, p300, and Hbo1, or of HDACs, such as HDAC1, HDAC2, HDAC3, and HDAC6,are associated with breast cancer progression or prognosis (85, 87). Histone lysine methylationcan cause either transcriptional activation or transcriptional repression, depending on the specific
Liang · Shang
residue and methylation state (mono-, di-, or trimethylation). The methyltransferase EZH2 cat-alyzes trimethylation of H3K27, and overexpression of EZH2 has a significant correlation withadvanced stages and poor prognosis of many types of cancer, including that of the breast. Ex-pression of other histone methyltransferases or demethylases is often altered in different stages ofbreast cancer (80, 87); such expression presumably affects global or local chromatin, alters geneexpression, and ultimately leads to tumor progression or drug resistance.
Although the underlying mechanisms for deregulated histone modifications in endocrine resis-
tance have yet to be defined, many laboratory and clinical studies have evaluated specific enzymeinhibitors in breast cancer treatment. Combining AZA and the HDAC inhibitor TSA can greatlyrestore ERα expression and sensitize ER-negative breast cancer cells to tamoxifen treatment (88).
A recent study demonstrates that addition of the HDAC inhibitor vorinostat (VPA) to ER-positivebreast cancer cells changes the normal response to tamoxifen from growth arrest to apoptotic celldeath. Thus, HDAC inhibitors may increase the efficacy of tamoxifen treatment (77). In this re-gard, the FDA has approved VPA for the treatment of cutaneous T cell lymphoma, and at least 80trials are under way to assess the clinical usefulness of other HDAC inhibitors in hematologicaland solid malignancies.
Theoretically, any cell that expresses ER could respond to estrogen and other ER modulators.
Sustained estrogen exposure or deregulated estrogen signaling is associated with many types ofcancer, including those of the breast, endometrium, ovary, prostate, lung, and colon. Althoughtamoxifen and AIs have been used mainly to treat breast cancer and certain types of endometrialcancer, we do not yet know whether these antiestrogen therapies could be used to treat othercancers. A recent report indicates that E2 stimulates ER-positive lung adenocarcinoma cell growthbut inhibits ER-negative lung adenocarcinoma cell growth (89). Whether interfering with estrogensignaling benefits ER-positive lung cancer patients remains to be determined. Developing newERα-specific SERMs is still one of the major strategies for treating tamoxifen-resistant breastcancer and other estrogen-related diseases. Increasing evidence indicates that changing the ratioof ERα/ERβ expression may play a pivotal role in tumor development and progression. Given thatERβ acts as a tumor suppressor to inhibit cell proliferation, cell growth, and angiogenesis, an ERβagonist could benefit ERβ-positive cancer patients. Future efforts to target ERβ offer an alternative
by Harvard University on 02/12/13. For personal use only.
approach to finding new ERα-specific SERMs. Finally, identifying global gene signatures thatpredict the antiestrogen responsiveness of specific tissues could better guide clinical applicationsof single and combined drug therapies in the fight against estrogen-responsive cancers.
Annu. Rev. Physiol. 2013.75:225-240. Downloaded from www.annualreviews.org
1. Estrogen is a well-established risk factor for many types of cancer, particularly breast and
endometrial cancer.
2. The primary mediators of estrogen action are estrogen receptor (ER)α and ERβ, which
function as ligand-induced transcription factors to regulate the expression of diversegenes.
3. The oncogenic effects of estrogen are due mainly to ERα-mediated transcriptional acti-
vation of genes that promote cell proliferation or alleviate apoptosis.
www.annualreviews.org • Estrogen and Cancer
4. Selective estrogen receptor modulators (SERMs) and aromatase inhibitors (AIs) are the
two main antiestrogen therapies.
5. Epigenetic factors contribute greatly to endocrine resistance in breast cancer treatment.
The authors are not aware of any affiliations, memberships, funding, or financial holdings thatmight be perceived as affecting the objectivity of this review.
Research in our lab was supported by grants (81130048 and 30921062 to Y.S.) from the NationalNatural Science Foundation of China and grants (973 Program: 2011CB504204 to J.L. and Y.S.)from the Ministry of Science and Technology of China.
1. Koos RD. 2011. Minireview: putting physiology back into estrogens' mechanism of action. Endocrinology
2. Shang Y. 2006. Molecular mechanisms of oestrogen and SERMs in endometrial carcinogenesis. Nat. Rev.
Cancer 6:360–68
3. Shang Y. 2007. Hormones and cancer. Cell Res. 17:277–794. Prentice RL, Anderson GL. 2008. The women's health initiative: lessons learned. Annu. Rev. Public Health
5. Auchus ML, Auchus RJ. 2012. Human steroid biosynthesis for the oncologist. J. Investig. Med. 60:495–
6. Miller WL, Auchus RJ. 2011. The molecular biology, biochemistry, and physiology of human steroido-
genesis and its disorders. Endocr. Rev. 32:81–151
7. Chumsri S, Howes T, Bao T, Sabnis G, Brodie A. 2011. Aromatase, aromatase inhibitors, and breast
cancer. J. Steroid Biochem. Mol. Biol. 125:13–22
8. Brueggemeier RW. 2001. Aromatase, aromatase inhibitors, and breast cancer. Am. J. Ther. 8:333–
by Harvard University on 02/12/13. For personal use only.
9. Cleary MP, Grossmann ME. 2009. Minireview: obesity and breast cancer: the estrogen connection.
Annu. Rev. Physiol. 2013.75:225-240. Downloaded from www.annualreviews.org
10. Wibowo E, Schellhammer P, Wassersug RJ. 2011. Role of estrogen in normal male function: clinical
implications for patients with prostate cancer on androgen deprivation therapy. J. Urol. 185:17–23
11. Folkerd EJ, Dowsett M. 2010. Influence of sex hormones on cancer progression. J. Clin. Oncol. 28:4038–
12. Jensen EV, Jacobson HI, Walf AA, Frye CA. 2010. Estrogen action: a historic perspective on the impli-
cations of considering alternative approaches. Physiol. Behav. 99:151–62
13. Heldring N, Pike A, Andersson S, Matthews J, Cheng G, et al. 2007. Estrogen receptors: How do they
signal and what are their targets. Physiol. Rev. 87:905–31
14. Bjornstrom L, Sjoberg M. 2005. Mechanisms of estrogen receptor signaling: convergence of genomic and
nongenomic actions on target genes. Mol. Endocrinol. 19:833–42
15. Hammes SR, Levin ER. 2011. Minireview: recent advances in extranuclear steroid receptor actions.
16. Thomas C, Gustafsson JA. 2011. The different roles of ER subtypes in cancer biology and therapy. Nat.
Rev. Cancer 11:597–608
Liang · Shang
17. Nilsson S, Koehler KF, Gustafsson JA. 2011. Development of subtype-selective oestrogen receptor-based
therapeutics. Nat. Rev. Drug Discov. 10:778–92
18. Kuiper GG, Enmark E, Pelto-Huikko M, Nilsson S, Gustafsson JA. 1996. Cloning of a novel receptor
expressed in rat prostate and ovary. Proc. Natl. Acad. Sci. USA 93:5925–30
19. Musgrove EA, Sutherland RL. 2009. Biological determinants of endocrine resistance in breast cancer.
Nat. Rev. Cancer 9:631–43
20. Leong H, Riby JE, Firestone GL, Bjeldanes LF. 2004. Potent ligand-independent estrogen receptor
activation by 3,3-diindolylmethane is mediated by cross talk between the protein kinase A and mitogen-activated protein kinase signaling pathways. Mol. Endocrinol. 18:291–302
21. Stender JD, Kim K, Charn TH, Komm B, Chang KC, et al. 2010. Genome-wide analysis of estro-
gen receptor α DNA binding and tethering mechanisms identifies Runx1 as a novel tethering factor inreceptor-mediated transcriptional activation. Mol. Cell. Biol. 30:3943–55
22. Jerry DJ, Dunphy KA, Hagen MJ. 2010. Estrogens, regulation of p53 and breast cancer risk: a balancing
act. Cell. Mol. Life Sci. 67:1017–23
23. Dupont S, Krust A, Gansmuller A, Dierich A, Chambon P, Mark M. 2000. Effect of single and compound
knockouts of estrogen receptors α (ERα) and β (ERβ) on mouse reproductive phenotypes. Development127:4277–91
24. Imamov O, Lopatkin NA, Gustafsson JA. 2004. Estrogen receptor β in prostate cancer. N. Engl. J. Med.
25. Forster C, Makela S, Warri A, Kietz S, Becker D, et al. 2002. Involvement of estrogen receptor β in
terminal differentiation of mammary gland epithelium. Proc. Natl. Acad. Sci. USA 99:15578–83
26. Antal MC, Krust A, Chambon P, Mark M. 2008. Sterility and absence of histopathological defects in
nonreproductive organs of a mouse ERβ-null mutant. Proc. Natl. Acad. Sci. USA 105:2433–38
27. Wang Z, Zhang X, Shen P, Loggie BW, Chang Y, Deuel TF. 2005. Identification, cloning, and expression
of human estrogen receptor-α36, a novel variant of human estrogen receptor-α66. Biochem. Biophys. Res.
Commun. 336:1023–27
28. Jordan VC, O'Malley BW. 2007. Selective estrogen-receptor modulators and antihormonal resistance in
breast cancer. J. Clin. Oncol. 25:5815–24
29. Li S, Shang Y. 2007. Regulation of SRC family coactivators by post-translational modifications.
Cell. Signal. 19:1101–12
30. Spencer TE, Jenster G, Burcin MM, Allis CD, Zhou J, et al. 1997. Steroid receptor coactivator-1 is a
histone acetyltransferase. Nature 389:194–98
31. Chen H, Lin RJ, Schiltz RL, Chakravarti D, Nash A, et al. 1997. Nuclear receptor coactivator ACTR is
a novel histone acetyltransferase and forms a multimeric activation complex with P/CAF and CBP/p300.
Cell 90:569–80
by Harvard University on 02/12/13. For personal use only.
32. Liang J, Zhang H, Zhang Y, Zhang Y, Shang Y. 2009. GAS, a new glutamate-rich protein, interacts
differentially with SRCs and is involved in oestrogen receptor function. EMBO Rep. 10:51–57
33. Tsai HW, Katzenellenbogen JA, Katzenellenbogen BS, Shupnik MA. 2004. Protein kinase A activation
of estrogen receptor α transcription does not require proteasome activity and protects the receptor from
Annu. Rev. Physiol. 2013.75:225-240. Downloaded from www.annualreviews.org
ligand-mediated degradation. Endocrinology 145:2730–38
34. Bunone G, Briand PA, Miksicek RJ, Picard D. 1996. Activation of the unliganded estrogen receptor by
EGF involves the MAP kinase pathway and direct phosphorylation. EMBO J. 15:2174–83
35. Wang C, Fu M, Angeletti RH, Siconolfi-Baez L, Reutens AT, et al. 2001. Direct acetylation of the
estrogen receptor α hinge region by p300 regulates transactivation and hormone sensitivity. J. Biol.
Chem. 276:18375–83
36. Berry NB, Fan M, Nephew KP. 2008. Estrogen receptor-α hinge-region lysines 302 and 303 regulate
receptor degradation by the proteasome. Mol. Endocrinol. 22:1535–51
37. Le Romancer M, Poulard C, Cohen P, Sentis S, Renoir JM, Corbo L. 2011. Cracking the estrogen
receptor's posttranslational code in breast tumors. Endocr. Rev. 32:597–622
38. Chen D, Ma H, Hong H, Koh SS, Huang SM, et al. 1999. Regulation of transcription by a protein
methyltransferase. Science 284:2174–77
39. Schurter BT, Koh SS, Chen D, Bunick GJ, Harp JM, et al. 2001. Methylation of histone H3 by coactivator-
associated arginine methyltransferase 1. Biochemistry 40:5747–56
www.annualreviews.org • Estrogen and Cancer
40. Chevillard-Briet M, Trouche D, Vandel L. 2002. Control of CBP co-activating activity by arginine
methylation. EMBO J. 21:5457–66
41. Naeem H, Cheng D, Zhao Q, Underhill C, Tini M, et al. 2007. The activity and stability of the tran-
scriptional coactivator p/CIP/SRC-3 are regulated by CARM1-dependent methylation. Mol. Cell. Biol.
27:120–34
42. Sims RJ 3rd, Rojas LA, Beck D, Bonasio R, Schuller R, et al. 2011. The C-terminal domain of RNA
polymerase II is modified by site-specific methylation. Science 332:99–103
43. Carascossa S, Dudek P, Cenni B, Briand PA, Picard D. 2010. CARM1 mediates the ligand-independent
and tamoxifen-resistant activation of the estrogen receptor α by cAMP. Genes Dev. 24:708–19
44. Shang Y, Hu X, DiRenzo J, Lazar MA, Brown M. 2000. Cofactor dynamics and sufficiency in estrogen
receptor-regulated transcription. Cell 103:843–52
45. Carroll JS, Meyer CA, Song J, Li W, Geistlinger TR, et al. 2006. Genome-wide analysis of estrogen
receptor binding sites. Nat. Genet. 38:1289–97
46. Carroll JS, Liu XS, Brodsky AS, Li W, Meyer CA, et al. 2005. Chromosome-wide mapping of estro-
gen receptor binding reveals long-range regulation requiring the forkhead protein FoxA1. Cell 122:33–43
47. Fullwood MJ, Liu MH, Pan YF, Liu J, Xu H, et al. 2009. An oestrogen-receptor-α-bound human chro-
matin interactome. Nature 462:58–64
48. Zhao C, Putnik M, Gustafsson J- ˚A, Dahlman-Wright K. 2009. Microarray analysis of altered gene ex-
pression in ERβ-overexpressing HEK293 cells. Endocrine 36:224–32
49. Musgrove EA, Caldon CE, Barraclough J, Stone A, Sutherland RL. 2011. Cyclin D as a therapeutic target
in cancer. Nat. Rev. Cancer 11:558–72
50. Caldon CE, Sutherland RL, Musgrove E. 2010. Cell cycle proteins in epithelial cell differentiation:
implications for breast cancer. Cell Cycle 9:1918–28
51. Butt AJ, McNeil CM, Musgrove EA, Sutherland RL. 2005. Downstream targets of growth factor and
oestrogen signalling and endocrine resistance: the potential roles of c-Myc, cyclin D1 and cyclin E.
Endocr. Relat. Cancer 12(Suppl. 1):47–59
52. Wang C, Mayer JA, Mazumdar A, Fertuck K, Kim H, et al. 2011. Estrogen induces c-myc gene expression
via an upstream enhancer activated by the estrogen receptor and the AP-1 transcription factor. Mol.
Endocrinol. 25:1527–38
53. Strom A, Hartman J, Foster JS, Kietz S, Wimalasena J, Gustafsson JA. 2004. Estrogen receptor β inhibits
17β-estradiol-stimulated proliferation of the breast cancer cell line T47D. Proc. Natl. Acad. Sci. USA101:1566–71
54. Gompel A, Somai S, Chaouat M, Kazem A, Kloosterboer HJ, et al. 2000. Hormonal regulation of apoptosis
in breast cells and tissues. Steroids 65:593–98
by Harvard University on 02/12/13. For personal use only.
55. Ariazi EA, Cunliffe HE, Lewis-Wambi JS, Slifker MJ, Willis AL, et al. 2011. Estrogen induces apopto-
sis in estrogen deprivation-resistant breast cancer through stress responses as identified by global geneexpression across time. Proc. Natl. Acad. Sci. USA 108:18879–86
Annu. Rev. Physiol. 2013.75:225-240. Downloaded from www.annualreviews.org
56. Lewis-Wambi JS, Jordan VC. 2009. Estrogen regulation of apoptosis: How can one hormone stimulate
and inhibit? Breast Cancer Res. 11:206
57. Vasconsuelo A, Pronsato L, Ronda AC, Boland R, Milanesi L. 2011. Role of 17β-estradiol and testosterone
in apoptosis. Steroids 76:1223–31
58. Miller VM, Duckles SP. 2008. Vascular actions of estrogens: functional implications. Pharmacol. Rev.
59. Bendrik C, Dabrosin C. 2009. Estradiol increases IL-8 secretion of normal human breast tissue and breast
cancer in vivo. J. Immunol. 182:371–78
60. Lindahl G, Saarinen N, Abrahamsson A, Dabrosin C. 2012. Tamoxifen, flaxseed, and the lignan entero-
lactone increase stroma- and cancer cell-derived IL-1Ra and decrease tumor angiogenesis in estrogen-dependent breast cancer. Cancer Res. 71:51–60
61. McCune K, Mehta R, Thorat MA, Badve S, Nakshatri H. 2010. Loss of ERα and FOXA1 expression in
a progression model of luminal type breast cancer: insights from PyMT transgenic mouse model. Oncol.
Rep. 24:1233–39
Liang · Shang
62. Wang X, Belguise K, Kersual N, Kirsch KH, Mineva ND, et al. 2007. Oestrogen signalling inhibits
invasive phenotype by repressing RelB and its target BCL2. Nat. Cell Biol. 9:470–78
63. Guttilla IK, Adams BD, White BA. 2012. ERα, microRNAs, and the epithelial-mesenchymal transition
in breast cancer. Trends Endocrinol. Metab. 23:73–82
64. Saha Roy S, Vadlamudi RK. 2012. Role of estrogen receptor signaling in breast cancer metastasis. Int. J.
Breast Cancer 2012:654698
65. Bai J, Uehara Y, Montell DJ. 2000. Regulation of invasive cell behavior by taiman, a Drosophila protein
related to AIB1, a steroid receptor coactivator amplified in breast cancer. Cell 103:1047–58
66. Yan J, Erdem H, Li R, Cai Y, Ayala G, et al. 2008. Steroid receptor coactivator-3/AIB1 promotes cell
migration and invasiveness through focal adhesion turnover and matrix metalloproteinase expression.
Cancer Res. 68:5460–68
67. Lydon JP, O'Malley BW. 2011. Minireview: steroid receptor coactivator-3: a multifarious coregulator in
mammary gland metastasis. Endocrinology 152:19–25
68. Griggs JJ, Somerfield MR, Anderson H, Henry NL, Hudis CA, et al. 2011. American Society of Clinical
Oncology endorsement of the cancer care Ontario practice guideline on adjuvant ovarian ablation inthe treatment of premenopausal women with early-stage invasive breast cancer. J. Clin. Oncol. 29:3939–42
69. Zhou WB, Ding Q, Chen L, Liu XA, Wang S. 2011. Toremifene is an effective and safe alternative to
tamoxifen in adjuvant endocrine therapy for breast cancer: results of four randomized trials. Breast CancerRes. Treat. 128:625–31
70. Wardell SE, Marks JR, McDonnell DP. 2011. The turnover of estrogen receptor α by the selective
estrogen receptor degrader (SERD) fulvestrant is a saturable process that is not required for antagonistefficacy. Biochem. Pharmacol. 82:122–30
71. Wittmann BM, Sherk A, McDonnell DP. 2007. Definition of functionally important mechanistic differ-
ences among selective estrogen receptor down-regulators. Cancer Res. 67:9549–60
72. 2006. STAR trial reports. Eur. J. Cancer 42:169473. McDonnell DP, Wardell SE. 2010. The molecular mechanisms underlying the pharmacological actions
of ER modulators: implications for new drug discovery in breast cancer. Curr. Opin. Pharmacol. 10:620–28
74. Shiau AK, Barstad D, Loria PM, Cheng L, Kushner PJ, et al. 1998. The structural basis of estro-
gen receptor/coactivator recognition and the antagonism of this interaction by tamoxifen. Cell 95:927–37
75. Shang Y, Brown M. 2002. Molecular determinants for the tissue specificity of SERMs. Science 295:2465–6876. Wu H, Chen Y, Liang J, Shi B, Wu G, et al. 2005. Hypomethylation-linked activation of PAX2 mediates
tamoxifen-stimulated endometrial carcinogenesis. Nature 438:981–87
by Harvard University on 02/12/13. For personal use only.
77. Thomas S, Thurn KT, Bicaku E, Marchion DC, Munster PN. 2011. Addition of a histone deacetylase
inhibitor redirects tamoxifen-treated breast cancer cells into apoptosis, which is opposed by the inductionof autophagy. Breast Cancer Res. Treat. 130:437–47
Annu. Rev. Physiol. 2013.75:225-240. Downloaded from www.annualreviews.org
78. Jordan VC. 2007. New insights into the metabolism of tamoxifen and its role in the treatment and
prevention of breast cancer. Steroids 72:829–42
79. Hoskins JM, Carey LA, McLeod HL. 2009. CYP2D6 and tamoxifen: DNA matters in breast cancer.
Nat. Rev. Cancer 9:576–86
80. Green KA, Carroll JS. 2007. Oestrogen-receptor-mediated transcription and the influence of co-factors
and chromatin state. Nat. Rev. Cancer 7:713–22
81. Burwinkel B, Wirtenberger M, Klaes R, Schmutzler RK, Grzybowska E, et al. 2005. Association of
NCOA3 polymorphisms with breast cancer risk. Clin. Cancer Res. 11:2169–74
82. Bergamaschi A, Katzenellenbogen BS. 2012. Tamoxifen downregulation of miR-451 increases 14-3-3ζ
and promotes breast cancer cell survival and endocrine resistance. Oncogene 31:39–47
83. Veeck J, Esteller M. 2010. Breast cancer epigenetics: from DNA methylation to microRNAs. J. Mammary
Gland Biol. Neoplasia 15:5–17
84. Kelly TK, De Carvalho DD, Jones PA. 2010. Epigenetic modifications as therapeutic targets.
Nat. Biotechnol. 28:1069–78
www.annualreviews.org • Estrogen and Cancer
85. Pathiraja TN, Stearns V, Oesterreich S. 2010. Epigenetic regulation in estrogen receptor positive breast
cancer—role in treatment response. J. Mammary Gland Biol. Neoplasia 15:35–47
86. Reis-Filho JS, Pusztai L. 2011. Gene expression profiling in breast cancer: classification, prognostication,
and prediction. Lancet 378:1812–23
87. Dalvai M, Bystricky K. 2010. The role of histone modifications and variants in regulating gene expression
in breast cancer. J. Mammary Gland Biol. Neoplasia 15:19–33
88. Badia E, Oliva J, Balaguer P, Cavailles V. 2007. Tamoxifen resistance and epigenetic modifications in
breast cancer cell lines. Curr. Med. Chem. 14:3035–45
89. Lin S, Lin CJ, Hsieh DP, Li LA. 2012. ERα phenotype, estrogen level, and benzo[a]pyrene exposure
modulate tumor growth and metabolism of lung adenocarcinoma cells. Lung Cancer 75:285–92
by Harvard University on 02/12/13. For personal use only.
Annu. Rev. Physiol. 2013.75:225-240. Downloaded from www.annualreviews.org
Liang · Shang
Source: http://www.leuiu.com/picture/article/2/fa/98/8c847c0b448d9d5dc006d802e41c/7fb58a6e-bc13-49a4-8078-dd90674ab0d3.pdf
Imaging an adapted dento-alveolar complex Ralf-Peter Herber1 , Justine Fong2, Seth A. Lucas1, Sunita P. Ho2* 1 Division of Orthodontics, Department of Orofacial Sciences, University of California, San Francisco, CA 94143, USA 2 Division of Biomaterials and Bioengineering, Department of Preventive and Restorative Dental Sciences, University of California San Francisco, San Francisco, CA 94143, USA
2014–2015 Student Injury and Sickness Insurance Plan for Northern Michigan University Who is eligible to enroll? All eligible registered students taking credit hours are required to enroll in the plan on a Hard Waiver basis. Eligible Dependents of students enrolled in the Student Health Insurance plan may participate in the plan on a voluntary basis. Eligible Dependents are the student's spouse and dependent children under 26 years of age.